Light (Li, Be, B) and volatile (F, Cl, H, C) elements
In the last years, much effort has been devoted by mineralogists and geochemists toward characterising the distribution of light elements in geologic materials. Light elements can play a significant role in rock-forming minerals (e.g., mica, amphibole, etc..).
The natural minerals to be used as reference materials are often markedly inhomogeneous, with compositional zoning or heavy contamination by micro-scale inclusions. The use of standards of composition appropriate to all samples to be analysed is not generally practicable for such complex materials, as for instance, tourmaline and mica. Therefore, in spite of the increasing demand for accurate analysis of such elements, in-situ investigation of Li, Be and B is still a challenge in routine analytical procedures.
We have developed a general SIMS procedure to measure Li, Be and B in silicates, starting from the fact that the reference samples to be used as standards for light elements are nowadays very scarce. The adoption of the "energy filtering" technique, generally used to remove molecular interferences, and therefore, in principle, not strictly necessary for the analysis of light elements, has revealed the key both to reduce matrix effects and improve measurement reproducibility. Such a development (Ottolini et al., 1993) represents a fundamental step for the application of SIMS in the analysis of light elements in minerals as well as in natural and synthetic glasses.
SIMS analyses, carried out on B in kornerupines showed the high level of accuracy achieved, ~ 3% rel. (Fig. 1).
Fig. 1- Comparison of B2O3 (wt%) contents in 32 crystals of kornerupine, determined by SIMS and structure refinement (SREF); the diagonal line is drawn with a slope of unit and is not a least squares line (Hawthorne et al., 1995a). |
The energy filtering was adopted also to reduce matrix effects on F/Si and Cl/Si ionisation. We showed, in particular, that the volatile species F and Cl can be quantitatively and efficiently analysed as positive secondary ions, in spite of their electronegative character. Cl is analysed as both 35Cl+ and 37Cl+ secondary ions. This allows for checking residual interferences (if any) at those mass numbers (Fig. 2).
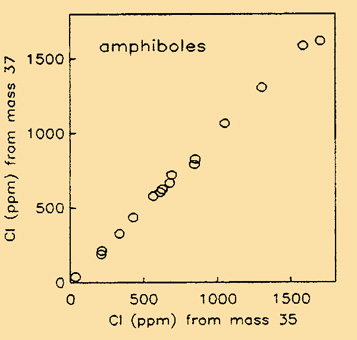
Fig. 2- Isotopic control of Cl results in amphiboles (Ottolini et al., 1994). |
As for F, its accurate and precise analysis cannot be considered as "routine" in the investigation of minerals. Electron microprobe analysis is limited by several factors: low peak intensity, effect of chemical bonding on the X-ray emission (which produces dramatic changes in peak shape and peak shifts), matrix effects related to X-ray absorption in the sample, and presence of interferences by higher-order lines of heavier elements. On the other hand, few SIMS data are available for F. The reason is due to the presence of matrix effects as well as to the lack of well-characterised reference samples that can be used for calibration.
We have carried out a comparative and systematic study in the analysis of fluorine in F-rich minerals, such as norbergite, chondrodite, clinohumite, fluoborite and topaz, analysed under a known crystallographic orientation (Ottolini et al., 2000; Camara & Ottolini, 2000; Ottolini & Camara, 2000) (Fig. 3). SIMS matrix effects and artefacts or limitations related to EMPA and SREF have been discussed, and an analytical protocol proposed for the analysis of high-F minerals.
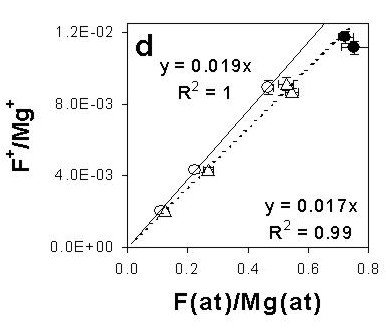
Fig. 3- Working curves F(Mg), obtained by plotting ion-intensity ratio F+/Mg+ vs. atomic concentration ratio F(at)/Mg(at). Concentrations from EMP analyses obtained at 7 keV with Area Peak Factors (APF) corrections. Symbols: open circles = "disoriented" humite-group samples; triangles = "oriented" humite-group samples; filled circles = fluoborite samples. Depending on orientation, the results define two ion yields, IY(F/Mg), differing by ~ 12%; in the most unfavourable cases, the difference among all IYs, including those of fluoborite, can be up to ~ 27% (Ottolini et al., 2000) |
The same approach, i.e., energy filtering + secondary positive ions, was successfully employed in the development of a quantitative analysis of C in rhyolitic glasses.
The flexibility of our SIMS procedure allow us to monitor volatile elements together with light, rare earth and other trace elements in the same selected microanalytical area. As an example, F and Cl, monitored with REE, Sr, Y, Zr, Cr, V, Sc and Ti have contributed to understanding the strain mechanisms at high temperature in gabbros from Ligurian ophiolites (Northern Apennine) (Tribuzio et al., 1995).
Further advancement in crystal chemical and geochemical work was achieved with the development of a SIMS procedure to quantify H in minerals and glasses (Ottolini et al., 1995). In particular, by detecting medium-, high-energy secondary ions we could:
- get a working curve valid over a wide compositional range (SiO2: 27-50 wt%)
- determine specific ion yields for rhyolitic glasses
- reduce H background in the secondary-ion mass spectra
An interesting application has involved the analysis of H in carlosturanite, a H2O-, Mg-rich, Si-poor, serpentine-like asbestiform mineral. The main results of our study are the following: i) a revisiting of its H2O content, ~ 13.50 wt% by SIMS, against ~ 17 wt% by TGA; ii) the extensibility of our SIMS procedures to the quantification of high amounts of H in low-silica minerals (Alberico & Ottolini, 1997).
A study carried out on silicates of various composition confirmed the key role of silica as well as Fe content in the ionisation of H (Ottolini & Hawthorne, 2001; Ottolini & Camara, 2001; Ottolini et al., 2002; Braga et al., 2003).
A model of H ionisation has been proposed in kornerupine, related to the Fe content of the matrix, and accurate analysis of H2O is feasible for any composition of this mineral by employing, preferentially, two standards. Moreover, such a procedure can be extended to silicates of different chemistry and structure by using a small number of standards and mathematical corrections on the ion yield (Ottolini & Hawthorne, 2001).
Our procedure for light and volatile elements has been applied and/or specialised for the analysis of tourmaline (rossmanite, elbaite, schorl, foitite, dravite, …) (Ottolini & Hawthorne, 1999; Selway et al., 1998; Aurisicchio et al., 1999a,b; Teertstra et al., 1999; Novák et al., 1999a; Camara et al., 2002), axinite (Andreozzi et al., 2000); cordierite (Malcherek et al., 2001), feldspar and borate (Cerný et al., 2002), sekaninaite (Cerný et al., 1997), staurolite (Hawthorne et al., 1993; Oberti et al., 1996), mica (Oberti et al., 1993; Liang et al., 1995; Cerný et al., 1995; Novák et al., 1999b), danalite (Nimis et al., 1996), amphibole (Hawthorne et al., 1993; Zanetti et al., 1994; Hawthorne et al., 1994; Hawthorne et al., 1995b; Hawthorne et al., 1996a,b; Zanetti et al., 1997; Hawthorne et al., 1998; Tiepolo et al., 1999; Zanetti et al., 2000; Hawthorne et al., 2000; Oberti et al., 2000; Hawthorne et al., 2001; Caballero et al., 2002; Oberti et al., 2003a,b; Oberti et al., 2004; Tait et al., 2005; Oberti et al., 2005), emerald, bazzite and milarite (Raade et al., 2004).
As an example, in case of axinite, SIMS investigations of B made it possible to show an inverse correlation with Si content, through a direct measurement of B [1.88 to 2.07 atoms per formula unit] (Andreozzi et al., 2000). The accuracy was calculated as ±2.5% rel. (Fig. 4).
Fig. 4- B content by SIMS vs. Si of studied axinites. Error bars: ± 1s for both B and Si. Horizontal and vertical dashed lines: stoichiometric values of 2 apfu (for B) and 8 apfu (for Si). Solid line with slope of –1 shows the ideal Si ® B substitution (Andreozzi et al., 2000). |
Our procedure has been also employed in the investigation of Li and B in mantle minerals (ol, cpx, opx and sp) of xenoliths (Ottolini & McDonough, 1996) and in such a case we could test its reliability in accurately quantifying extremely low contents of Li (at ppm or sub ppm level) and B (in the range: few ppb-ppm).
The concentration of B in the mantle has been a matter of debate for a long time. An estimate of 0.1 ppm B seems to be consistent as a source for non-arc basalts, but such data have not been directly confirmed. Li and B literature contents for peridotites are derived from samples whose non- metasomatized character has not been established for both elements, due to the lack of a comprehensive metasomatism model for Li and B.
We have looked at two groups of mantle rocks, with and without clear compositional evidence that they are metasomatized. We proposed a diagnostic diagram based on (Ce/B) vs. (Li/Yb) as measured by SIMS in peridotite clinopyroxenes, which is useful in identifying metasomatized samples. After discovering samples with no metasomatic alteration, which are considered representative of the normal mantle, we derived for each mantle mineral phase (ol, opx, cpx and sp) the Mg#, Li and B partial-melting evolution trends. Additionally, considering that the “normal” mantle rocks have evolved through partial melting only, we assessed Li and B contents in the parental mantle of our samples, and assumed that the calculated values (1.6–1.8 ppm Li and 0.07–0.10 ppm B) are representative of the contents in MORB mantle sources (Ottolini et al., 2004).
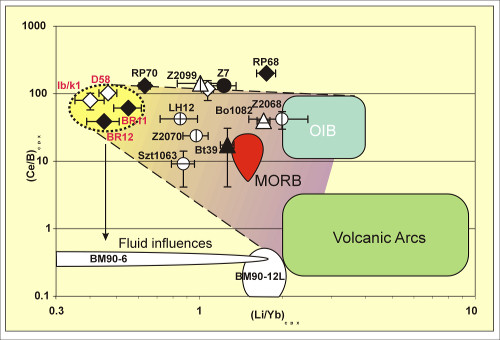
Fig. 5- Discriminating diagrams for Li and B metasomatic evolution in cpx. The fields enclosed in dotted lines represent the limit of the Sub Continental Depleted Mantle (SCDM). The samples whose heterogeneity is high are plotted as elliptical fields enclosing the average value for all measures, and the corresponding standard error. The fluid influence is strongly marked by the low (Ce/B)cpx exhibited by samples BM90-6 and BM90-12L (from Balmuccia, western Alps, Italy). The dashed lines limit the domain plotting samples compatible with direct influence of any kind of magma (OIB, MORB and Volcanic Arc) (Ottolini et al., 2004). |
Boron and chlorine cycling was investigated in the subducted hydrous oceanic mantle. The data indicate a depth-related decrease of Cl and B in bulk rocks and minerals, and point to their partitioning in the evolved fluids (Scambelluri et al., 2002; Scambelluri et al., 2004).
Our procedure can be used also in the investigation of natural glasses (Wulff-Pedersen et al., 1999), to estimate H content in experimental charges (Freda et al., 2001; Baker et al., 2002). It has been also extended to complex matrixes, such as REE minerals (See Complex Matrixes).
A comprehensive investigation of matrix effects as related to structure and chemistry, and ensuing improvement in the SIMS analysis of light and volatile elements have been carried out on a wide set of the most important rock-forming minerals (Ottolini et al., 2002).
The first example (at our knowledge) of a FTIR calibration for H2O in a complex mineral matrix based on a SIMS working curve -used as reference- (see Fig. 6) was recently obtained in a suite of vesuvianite samples from the volcanic ejecta (skarn or syenites) from Latium (Italy) (Bellatreccia et al., 2005a).
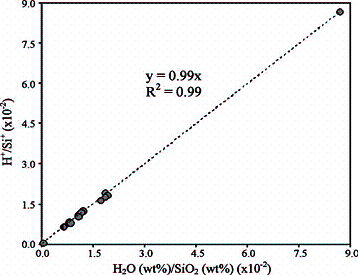
Fig. 6- Ratios of the secondary-ion currents for H and Si, H+/Si+, derived from 1H+/30Si+ and corrected for isotope abundance vs. H2O(wt%, SIMS) / SiO2(wt%, EMPA) (average values) for the studied vesuvianite samples and the elbaite standard used. |
The resulting integrated molar absorption FTIR coefficient for vesuvianite is ei =100.000± 2.000 l mol-1 cm-2, which is in excellent agreement with the ei values available from literature (see Fig. 7).
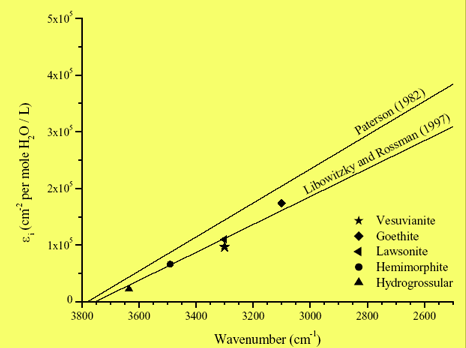
Fig. 7- The ei value derived for vesuvianite (Bellatreccia et al., 2005a) plotted on the IR calibration curves of Paterson (1982) and Libowitzky and Rossman (1997). The points representing lawsonite, a sorosilicate which has a spectrum similar to that of vesuvianite (Libowitzky and Rossman, 1996), hemimorphite (Libowitzky and Rossmann, 1997), goethite (Libowitzky, 1996) and hydrogrossular (Rossmann and Aines, 1991) are also shown for comparison. |
The relationship between the OH content (apfu) measured by SIMS and that derived by FTIR for all samples is shown in Fig. 8 as a function of the Mg (apfu) determined by EMPA. There is an excellent linear trend and a well-defined negative correlation between OH and Mg.
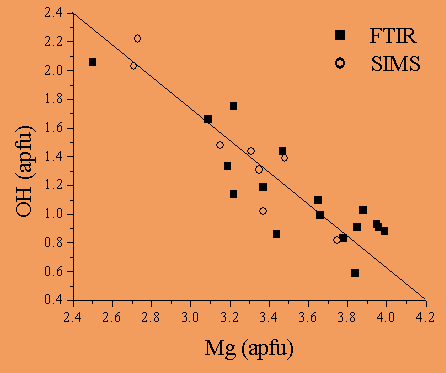
Fig. 8- Relationship between the OH content (apfu) determined by SIMS (open circle) and FTIR (filled square) versus Mg (apfu) by EMPA for all samples. The solid line is a guide for the eye (Bellatreccia et al., 2005a). This plot testifies the internal consistency of the calibration curves SIMS + FTIR, and provides further experimental evidence for the the incorporation of B into B-bearing vesuvianites via the MgBAl-1H-2 substitutional vector, in accord with Groat et al. (1992, 1994). |
A recent experimental study examines the combined effects of different piston cylinder assemblies and presaturated/unconditioned AuPd capsules on the oxygen fugacity (fO2) and the capacity to maintain constant H2O-contents in H2O undersaturated Fe-bearing basaltic system (Kägi et al., 2005). Fig. 9 shows an entire cross-section of an experimental charge containing the two capsules, quenched glasses, olivine and cpx crystals, and the SIMS spots in the inner and outer capsule.
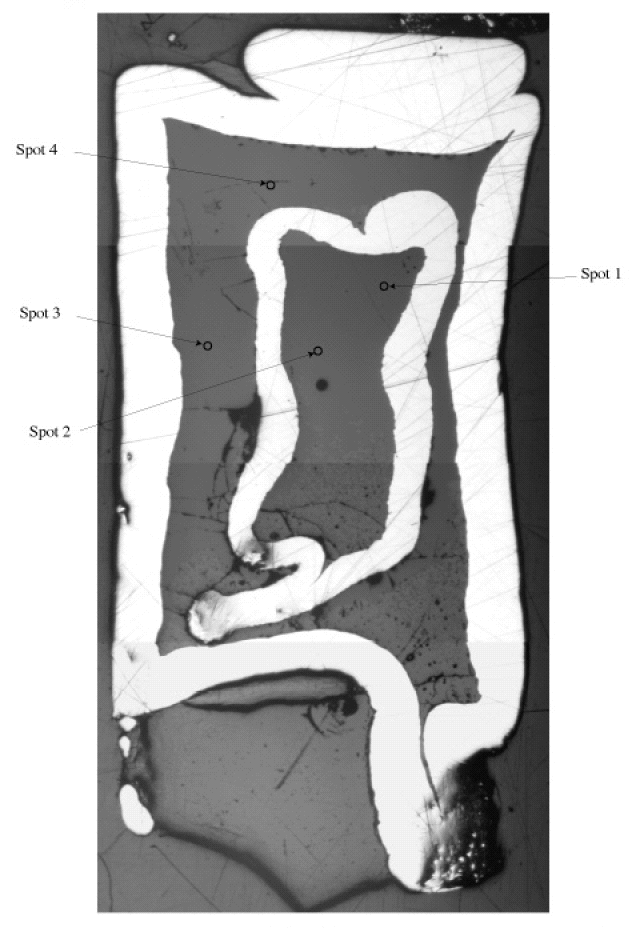
Fig. 9- (Kägi et al., 2005) |
The H2O contents of all glasses vary between 2.99 and 3.97 wt.% H2O with very narrow ranges for each individual glass not exceeding ±0.13 wt% H2O. The difference between the measured and calculated H2O content resulted to be on the same order as the uncertainty from SIMS measurements < 10 % rel., typically 3-5% rel. The conditions of this study are comparable to estimates of fO2 during the crystallization of natural calc-alkaline magmas (Kägi et al., 2005).
The H content was investigated at the ion microprobe in a mica named oxykinoshitalite. Our sample has very high contents of Ti (0.76 apfu) and Fe3+ (0.57 apfu) and very low content of Mn2+. The low contents of H2O (0.55 wt%) and F (0.91 wt%), and the dominance of O atoms at the O(4) site indicate that oxykinoshitalite from Fernando-de-Norohna Island (Brazil) is a new mineral species (Kogarko et al., 2005).
Different trioctahedral 1M mica populations occur at Mt. Vulture. The micas are phlogopite-annite solid solutions with a minor component of brittle micas (kinoshitalite-ferrokinoshitalite solid solutions) and can be distinguished on the basis of the XMg ratio and on the relative content of Ti and F. A selected suite was investigated by SREF, EMPA and SIMS. All the analyzed sample have (OH + F) ~ 2 a.p.f.u. so that the oxy-type of substitution plays a very minor role. Chemical and structural details show remarkable variations between the red (Ti- and F-rich) and green micas and within the green (Ti- and F-poor) micas. Complex processes, such as magma mixing and/or interactions with aqueous fluids have been invoked for that (see Mesto et al., 2006 for details).
Olivine + clinopyroxene ± amphibole cumulates have been widely documented in island arc settings and may constitute a significant portion of the lowermost arc crust. Because of the low melting temperature of amphibole (~ 1100° C), such cumulates could melt during intrusion of primary mantle magmas. We have experimentally (piston-cylinder, 0.5–1.0 GPa, 1200–1350°C, Pt–graphite capsules) investigated the melting behaviour of a model amphibole–olivine–clinopyroxene rock, to assess the possible role of such cumulates in island arc magma genesis. Water concentrations in some glasses were determined using our Cameca IMS 4f ion microprobe. The experimental results are described in Médard et al., 2006.
H, B and F were quantified by SIMS in a suite of minerals using, in turn, Al and Ca (instead of the more used Si) as the reference element for the matrix. The accurate results obtained indicate that these two elements may be used successfully in the quantification procedure. Moreover, on the basis of our experience and on literature data, it seems that IY(Element/Si) is sensitive to changes which occur with time and with differing ion-bombardment conditions. Variations in ion yield are also dependent on changes in beam density and focusing across the sample during analysis. Using Al or Ca instead of Si, the ion yields for light elements in silicates seem to be more stable with time and less sensitive to instrumental conditions (Ottolini et al., 2006).
In recent years there has been an increasing number of applications of micro-analytical techniques, with an ensuing request of well-characterised calibration standards. Such a request is particularly critical to SIMS due to the existence of matrix effects in the ion micro-probe analysis.
We have developed a technique to prepare a reference glassy sample starting from a powdered Standard Reference Material, represented by the basaltic rock JB-2 (Geological Survey of Japan), and reported the results of EMPA and SIMS investigations in the resulting glass. SIMS data on Li and B agree with the Li and B concentrations actually proposed as “preferred” values in the SRM JB-2, testifying that the whole composition of the starting material was not altered by the fusion process for the elements here investigated, except for the outermost material of the charge, that shall be discarded in the analytical work (Le Fevre & Ottolini, 2006).
This approach can be usefully adopted for the preparation of various reference silicates for Li and B elemental analysis thus overcoming the lack of certified solid standards for SIMS investigation. The method offers new potentialities also in the standardisation of silicate materials for Li and B isotope analysis provided that the final isotope composition is checked with an independent technique to exclude fractionation effects related to powder fusion.
A formidable task has been the SIMS analysis of B and other light and volatile elements in the suite of vesuvianite samples (Bellatreccia et al.,2006) owing to an extreme (micron-scale) inhomogeneity related to crystal zoning (see Fig. 10).
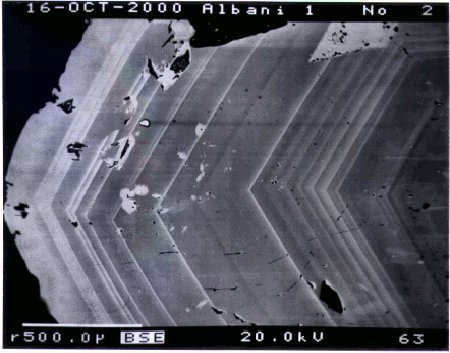
Fig. 10- Fig. 10- Backscattered electron image of the vesuvianite sample 44FB showing the fine-scale chemical zoning (Bellatreccia et al., 2006) |
Fig. 11 shows that there is a well-defined linear relation between the B2O3 content by SIMS and the measured absorbance of the B-O triplet of bands in the 1250-1450 cm-1 range.
The regression line through the experimental data can thus be used as a calibration for the quantitative measurement of boron in the samples: ai=34000±1400·B2O3 (wt%), where ai is the integral absorbance measured on the oriented [001] section along the e+w directions using polarised IR light. To our knowledge, this study represent the first calibration based on SIMS data for the quantitative analysis of boron in minerals by FTIR spectroscopy.
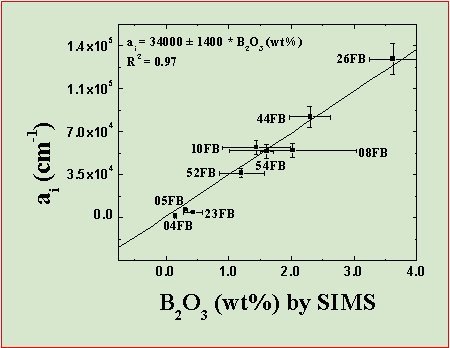
Fig. 11- Relationship between the B2O3 content (wt%) by SIMS and the FTIR integrated absorbance, ai for the bands in the 1200-1450 cm-1 region. The error bars on SIMS data represent the coefficient of variation CV (± 1σ) related to the sample inhomogeneity; the error for ai are estimated at 10% of the measured absorbance (Bellatreccia et al., 2006). |
Geochemical data on trace elements obtained by SIMS show that peridotites from Finero massif are characterised by different geochemical signatures which can be grouped in two major types based on LLE, LILE, REE and HFSE contents of cpx (see Fig. 12, 13). This result indicates that these peridotites experienced contrasted metasomatic overprints. In particular, the apatite-bearing peridotites seem to be mainly influenced by mantle-derived agents, whereas the apatite-free rocks underwent the addition of crust-derived components (Raffone et al.,2006).
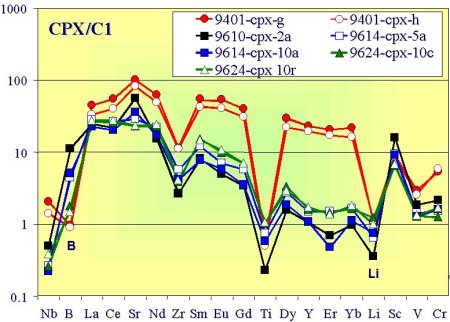
Fig. 12- Trace-element patterns of clinopyroxenes from the studied pyroxenite-peridotite sections normalized to C1 Chondrite composition. Circles are for apatite-bearing samples, squares for apatite-free peridotite samples, and triangles for pyroxenite |
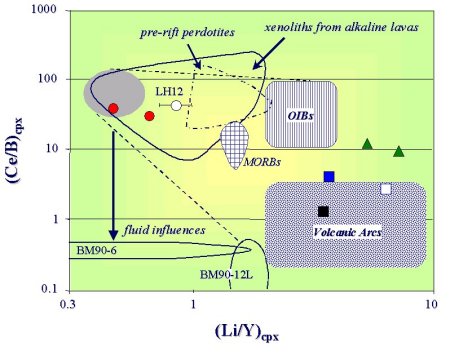
Fig. 13- Ce/B vs. Li/Yb ratios for Li and B metasomatic evolution in cpx. The field enclosed in the shaded area represents the limit of the sub-continental depleted mantle. The dashed lines limit the domain plotting samples compatible with direct influence of any kind of magma: Oceanic Island Basalt (OIB), Middle Oceanic Ridge Basalt (MORB) and Volcanic Arc. Black circles: apatite-bearing peridotite; open and filled squares: apatite-free peridotites; open triangles: pyroxenite sample (Raffone et al., 2006). |
The identification of provenance and working places of gemstones decorating archaeological jewels captured the attention of archaeologists and scientists in all the times. The attempt to characterize archaeological emeralds through their chemical composition, i.e., on the basis of the contents of their major elements, has given so far no valid results due to the impossibility to discriminate among different source deposits. The analysis of trace elements by SIMS represents a new reading key to approach the fluid-rock interaction which allows for the emerald growth. The presence and enrichment of some trace element (see Fig. 14) integrated with major and minor constituents revealed to be very promising in suggesting genetic models (Aurisicchio et al., 2007).
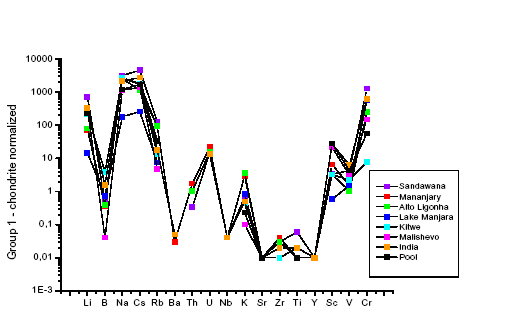
Fig. 14- Chondrite normalized (McDonough & Sun, 1995) trace-element patterns of the archaeological emeralds in study obtained by means of the ion microprobe at CNR-IGG (Pavia). |
Fluorophlogopite, a new F-dominant mineral of the mica group, was found at Monte Calvario, Biancavilla, lower southwestern flanks of Mt Etna volcano (Catania, Sicily, Italy) (Gianfagna et al., 2007). It was formed by metasomatism of the original lava rocks from very hot fluid enriched in F, Cl, and other incompatible elements. The new mineral fluorophlogopite and its name were approved by IMA-CNMMN (2006/011). SIMS measurements at CNR-IGG (Pavia) allowed to estimate light elements (Li2O = 0.30(1) and H2O = 0.16(2) wt%) and fluorine content (F = 8.69(24) wt%). From a geo-volcanological point of view the finding of the fluorophlogopite in the volcanic products of Biancavilla is further evidence that metasomatic processes affected the pre-existing benmoreitic rocks of the Etnean volcanic area. The presence of the fluorophlogopite in association with the fluoro-edenite is also consistent with this hypothesis.
The crystal chemistry of red phlogopite from Mt. Vulture (Italy) ignimbrites has been studied by electron microprobe, secondary ion mass spectrometry (SIMS), single crystal structural investigation and Fourier transform infrared (FTIR) spectroscopy. The analysed phlogopite has Fe/(Fe + Mg) = 0.35, TiO2 (wt%): 2.8–5.0 and H2O: 1.24–3.37 wt%. Infrared spectra revealed the presence of bandsdue to the NH4+ and H2O stretching and bending vibrations. The samples belong to the 1 M polytype. The bimodal behaviour of several structural parameters allows red micas to be clustered into two distinct groups:
K+ ↔ NH4+, H2O and M3+-vacancy substitutions dominate in the first group;
M3+,4+-oxy, in the second group.
The main result is that quantitative analysis of hydrogen (via SIMS) together with the characterization of the local environment of the anionic site (via FTIR) are fundamental in assessing the correct structural formula and the substitution mechanisms in micas (Scordari et al., 2008).
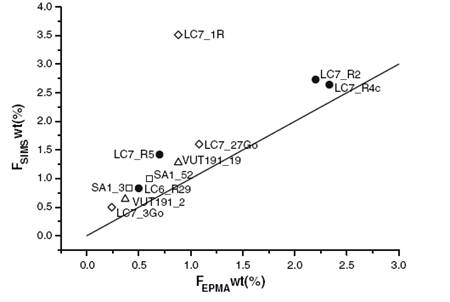
Fig. 15- Comparison between F data by SIMS and by EMPA in the set of micas from Vulture, showing that F from EMPA is generally under-estimated in this matrix (full circles red micas, this work: Scordari et al., 2008). Open symbols are literature data: Squares (Scordari et al. 2006), diamonds green and red micas (Mesto et al. 2006); triangles brown micas (Matarrese et al., 2007). |
In spite of the importance of chlorine as a geochemical tracer, Cl data on mantle rocks are nowadays rather scarce mainly due to analytical difficulties. Techniques involving sample dissolution and/or fusion as well as non-destructive bulk techniques are used for Cl determination in silicates but they need rather high amounts of material, which, in most cases, hampers the investigation of this element in single minerals. The SIMS technique that affords for low detection limits over a large mass range and a micron-scale spatial resolution represents a powerful tool to address some of the main questions still open about Cl behaviour in the Earth mantle. In-situ data for Cl were obtained on upper-mantle peridotites and xenoliths affected by various metasomatic processes, using a SIMS procedure optimised to quantify very low (ppm level) Cl concentration. We investigated the inter- and intra-mineral phase Cl distribution also in relation with other important geochemical tracers, such as B, Li, REE, Zr, Nb,... The analysis of Cl by SIMS shows to be a valid tool to get insights into the petrogenetic history of the rock (Ottolini & Le Fevre, 2008).
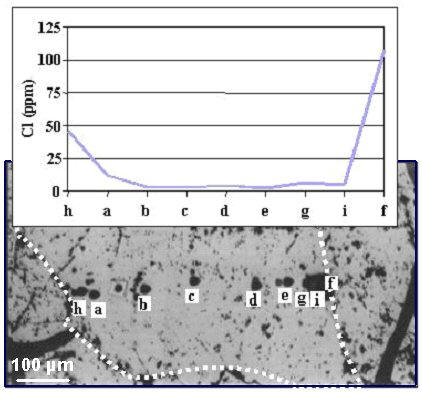
Fig. 16- Reflected-light optical micrographs of opx in BM90-6 (Balmuccia, Western Alps, Italy) indicating the SIMS spots and the corresponding Cl contents (ppm). Opx reacted in the presence of a metasomatic agent by Cl incorporation which seems to follow a diffusive law. The Cl enrichment is higher along the rim of the crystal and testifies that the metasomatic agent diffused along the grain boundaries (sketched by white dots in the micrographs) with as high as 108 ppm Cl (Ottolini & Le Févre, 2008). |
The combination of analytical methods used by Matarrese et al. (2008) has shown that volcanic micas (Mt. Vulture, Italy) are able to provide information related to their geological history even in complex cases such as those considered in the paper, where the micas are characterized by notable chemical variability. However, other than complete chemical and structural analysis, the use of single-crystal techniques, both for hydrogen determinations (such as SIMS) and for Fe speciation (such as micro-XANES or other element-sensitive techniques) could help to shed light into the uncertainties related to the results of the bulk analytical methods.
We have investigated by Secondary Ion Mass Spectrometry, at our knowledge for the first time, the Li, B and Cl contents on single minerals represented by plagioclase (plg) and clinopyroxene (cpx) of three selected drill-cuttings, one unaltered lava and one core-sample from wells RN-17 and RN-19, respectively, both wells being located in the Reykjanes high-T hydrothermal system, SW Iceland. This research has been funded to Luisa Ottolini by CNR grants (year 2005): RSTL: Study of basaltic crust of Iceland (International Deep Drilling Project, IDDP).
Fig. 17- Reflected-light optical micrographs of portions of cuttings RN-17-1100. Note SIMS craters after bombardments and the high heterogeneity at small scale |
Moreover, the comparison of Li (six samples) and B (six samples) whole rock data from Reykjanes geothermal system with those from literature shows that our samples seem to be mainly affected by seawater hydrothermal alteration. In the most B-enriched cutting RN-17-2150 a positive correlation occurs between B whole rock and Cl contents in the mineral phases, whereas, also due to the few available data, no systematic relationship seems to exist between the depth of well-drilling and the intensity of hydrothermal alteration. The discrepancy between the B concentrations in the whole rock and mineral phases (cpx and plg) could be related to high partitioning of boron in hydrothermally related secondary phases, such as epidote. As far as Cl is concerned, on the basis of the preliminary data so far obtained on single mineral phases, amphibole seems to be the main host for this element. Fracture zones in addition are responsible for local Cl enrichment (Raffone et al.,2008).
Despite the growing interest for Li and B as geochemical tracers, especially for material transfer from subducting slabs to overlying peridotites, little is known about the behaviour of these two elements during partial melting of mantle sources. In particular, mineral/melt partition coefficients for B and to a lesser extent Li are still a matter of debate. We re-equilibrated a synthetic basalt doped with ~ 10 ppm B and ~ 6 ppm Li with an olivine powder from a spinel lherzolite xenolith at 1 GPa–1330°C, and we analyzed Li and B in the run products by SIMS (Ottolini et al., 2009).
In our experiment, B behaved as a highly incompatible element, and Li as a moderately incompatible element (see Table below). Our partition coefficients for Li are in good agreement with previous determinations. In the case of B, our partition coefficients are equal within error to those reported by Brenan et al. (1998) for all the mineral phases analysed, but are lower than other coefficients from literature for some of the phases (up to 5 times for cpx). Our measurements complement the data set of D’s for modelling partial melting of the upper mantle and basalt generation, and confirm that, in this context, B is more incompatible than previously anticipated (Ottolini et al., 2009).
Dmin/melt | Li | Range | B | Range |
ol | 0.427 | 0.418-0.436 | 0.008 | 0.004-0.013 |
opx | 0.211 | 0.167-0.256 | 0.024 | 0.015-0.033 |
cpx | 0.246 | 0.229-0.264 | 0.041 | 0.021-0.061 |
Dmin/min | ||||
ol/opx | 2.02 | 0.33 | ||
ol/cpx | 1.74 | 0.20 | ||
cpx/opx | 1.17 | 1.71 |
The SIMS technique was used in the investigation of a H-poor and Fe-rich biotite from volcanic dacite rocks of Džep, Serbia, showing a syntactic texture of disordered 1Mr-n(120°), highly faulted 2M1, and ordered 3T sequences. This is the first report of 3T long-range-ordered stacking with a unique interlayer composition in a Li-poor trioctahedral mica (for details, see Fregola et al., 2009).
A SIMS analytical procedure to quantify extremely low H2O contents in Transantarctic Mountain microtektites has been recently developed at CNR-IGG, Pavia. The H2O content ranges from 28 to 206 µg/g. The lowest values were observed in the high-magnesium microtektites (28–44 µg/g) (Fig. 18A). A positive correlation with SiO2 (wt%) is observed (Fig. 18B). Transantarctic Mountain microtektites are essentially dry and have water contents similar to that of the Australasian microtektites, which ranges from <20 to 246 µg/g; moreover, a comparison with the limited literature database for macroscopic tektites from the Cenozoic tektite strewn fields reveals that the H2O content of the Trans. Mount. microtektites is within the range of tektites, 20 to 300 µg/g (see Folco et al., 2009 for details).
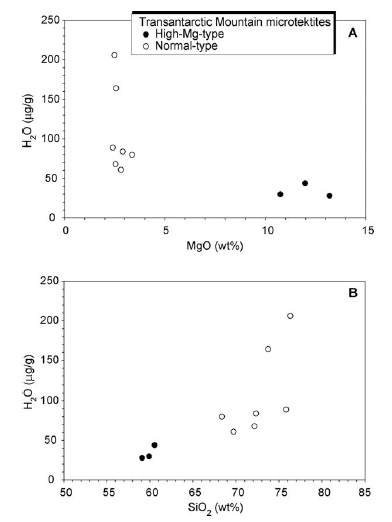
Fig. 18- H2O content (µg/g), obtained by SIMS, versus MgO (wt%) (A), and SiO2 (wt%) (B), for Transantarctic Mountain microtektites (subdivided into normal and high-Mg types) (Folco et al., 2009). |
The study by Alletti et al. (2009) mainly aimed at investigating the distribution of chlorine between a basaltic melt from Mt. Etna and a coexisting aqueous fluid phase (or phases) at different pressures and redox conditions for H2O–Cl and H2O–CO2–Cl fluids. Most of the experiments were done at chloride-brine-undersaturated conditions. The results of this experimental studies can significantly contribute to our understanding of chlorine behavior in basaltic magmas. In Fig. 19, concentrations of H2O, as obtained by our ion microprobe, versus Cl in the final melts are reported.
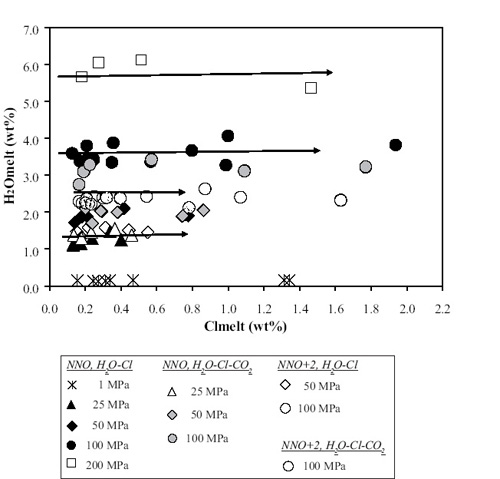
Fig. 19- Fig. 19- Concentrations of H2O, obtained by SIMS, versus Cl in the final melts. H2O content does not vary as Cl increases. Following Webster et al. (1999), and considering that most of our experiments were at initial Cl concentrations below brine saturation, this suggests that our experiments fall in the P–T–X region where only a hydrous vapor or fluid and melt were stable (Alletti et al., 2009). |
A full characterization of micas requires complete chemical analysis, including the determination of light elements, combined with Mössbauer spectroscopy or any technique suitable for the determination of ferric and ferrous iron contents, and crystal structural analysis (SCXRD). In this context, the SIMS technique is essential for a deeper understanding of the crystal chemistry of mica owing to its capability of precise and accurate quantification of light elements, i.e., H, Li, B, F, ... The role of SIMS has been outlined in the investigation of the complex crystal structure of mica, with new data on a set of volcanic samples, and their comparison with those from literature on trioctahedral micas from volcanic areas of Southern Italy. The importance of SIMS micro-spot (in-situ) analysis is emphasized as a modern approach to gain insight into physico-chemical processes that affect grain-to-grain or intra-grain chemical variability in complex mineral structures (Ottolini et al., 2010).
The light lithophile (Li, Be and B) and halogen (F, Cl) elements are powerful tracers of fluid transfer due to their mobility during high temperature hydrothermal processes and metamorphic devolatilization. Moreover, although a great deal of studies have been carried out on these elements in whole rock and minerals of altered rocks from divergent and convergent plate margins, an inventory for mineral phases from the altered Icelandic oceanic crust is still incomplete.
In the paper by Raffone et al. (2010) we report the results of in situ EPMA and SIMS investigations on variously altered magmatic (plagioclase and clinopyroxene) and hydrothermal phases (amphibole and epidote) from selected cuttings drilled at different depths (400–3000 m) of the well RN-17, Reykjanes geothermal system (SW Iceland). Our SIMS data on epidote have shown that alteration beneath Reykjanes has been more efficient in the shallow and intermediate cuttings, while whole rock data on boron isotope composition have revealed that the alteration has been caused firstly by d11B-poor fluids and successively by d11B-rich seawater-hydrothermal fluids.
Pertsevite-(OH), end-member formula Mg2(BO3)(OH), is a new mineral found in a ludwigite-kotoite magnesian skarn from the Snezhnoye deposit in Sakha-Yakutia Republic, Russia. The Commission on New Minerals, Nomenclature and Classification, IMA (IMA 2008-060) has approved the mineral and the mineral name. Moreover, the Chairman of the CNMNC agreed to renaming pertsevite to pertsevite-(F). The two minerals constitute the pertsevite series with the general formula Mg2(BO3)1–x (SiO4)x(OH,F)1–x, where x = 0.1–0.3. Ion microprobe analyses of pertsevite-(OH) using secondary ion mass spectrometry gave B and F data consistent with B and F contents determined by electron microprobe analysis (EMPA).
For the quantification of H in pertsevite-(OH), matrix-matched standards for SIMS calibration were not available. Nevertheless, we defined the level of water concentration in the pertsevite-(OH) sample set by calibrating with well-characterized low-silica silicate standards and then by applying mathematical corrections on the ion yield (IY). Similar values for water -on the order of ~ 3 wt% H2O- were obtained by using another approach, i.e., by calculating the best fit between the experimental ion yields IY(H/Mg), derived from two tourmaline standards and a pertsevite-(OH) grain of the set vs. their MgO (wt%) content. For the last sample, we used its H2O as derived from charge-balance calculations.
Both SIMS approaches confirmed an OH/F ratio > 1 in pertsevite-(OH), in accord with OH contents calculated from EMPA data (see Galuskina et al., 2010 for details).
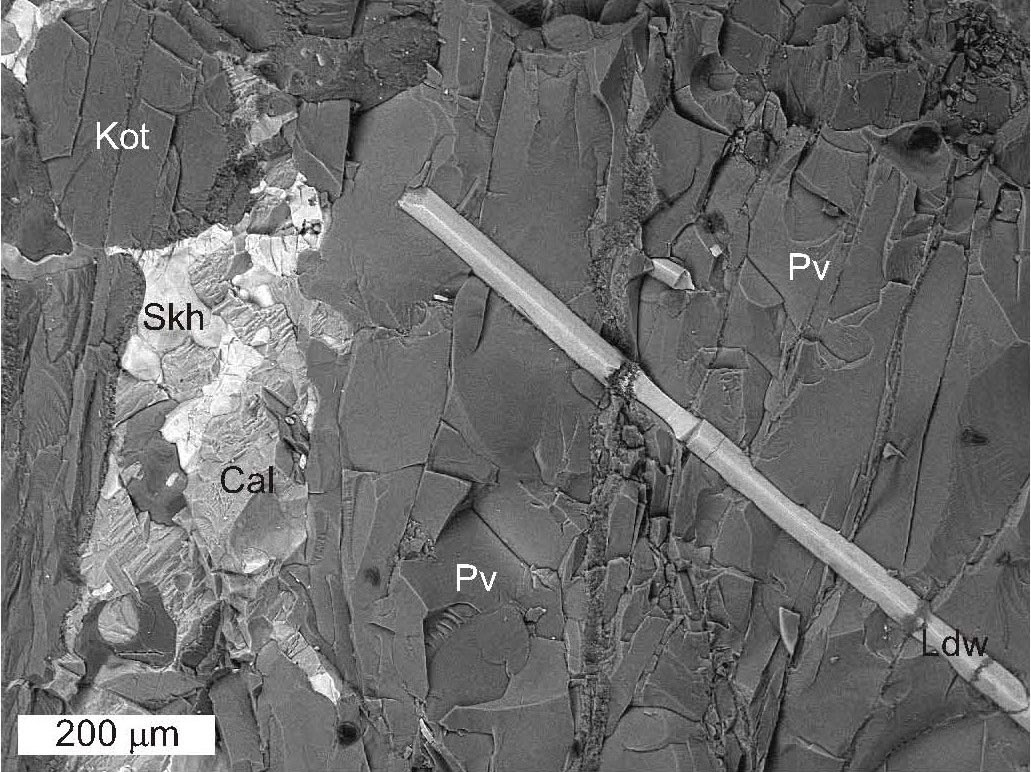
Fig. 20- Pertsevite-(OH) (Pv) associated with ludwigite (Ldw), kotoite (Kot), REE-bearing sakhaite (Skh), calcite (Cal), BSE image, low vacuum, 0.3 Torr (see Galuskina et al., 2010). |
The SIMS technique has been recently employed in the study of the complex crystal chemistry of dark blue aquamarine (Yukon Territory, Canada). Its 5.39 wt.% FeO in the darkest material is among the highest Fe concentrations known for true beryl (see Groat et al., 2010 for details).
Selected phlogopite flakes from Mt. Vulture in southern Italy were studied using a combination of single-crystal techniques: electron microprobe analysis (EMPA), secondary ion mass spectrometry (SIMS), single-crystal X-ray diffraction (SCXRD) and micro-X-ray absorption near-edge spectroscopy (XANES). SIMS analysis showed H2O (wt%) = 1.81-3.30, F (wt%) = 0.44-1.29, and Li2O (wt%) = 0.001-0.027. The intra single-crystal chemical variability for major/minor elements (Mg, Fe, Al, Ba, Ti and K) was found particularly significant for samples VUT191_11 and PG5_1. SIMS data relative to crystals VUT187_24, VUT191_10, VUT191_11 and VUT187_28 showed a noteworthy variation in the concentrations of some light elements (H, Li and F) with coefficient of variation CV (as 1s%) up to ~18% for H2O. The combination of EMPA, SIMS and micro-XANES resulted in the characterization of the samples at a comparable spatial scale. By means of in-situ data and the results of crystallographic investigations, the occurrence of different relative amounts of M3+-oxy (VIM2+ + (OH)- « VIM3+ + O2- + ½ H2) , Ti-oxy substitutions (VIM2+ + 2(OH)- « VITi4+ + 2O2- + H2 ) and Ti-vacancy (☐) substitution (2 VIM2+ « VITi4+ + VI☐) was ascertained for the studied samples (Scordari et al., 2010).
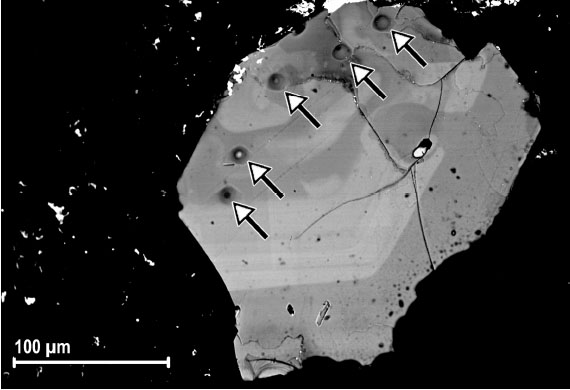
Fig. 21- SEM-BSE image of sample VUT191_11 after removal of Pt-coating used for ion-probe analysis. The SIMS craters are indicated by the arrows. The contrast due to chemical inhomogeneity is clearly visible (Scordari et al., 2010) |
Together with single crystal X-ray methods and electron microprobe analysis, ion probe was used to characterize fluor-dravite -NaMg3Al6Si6O18(BO3)3(OH)3F- a new mineral of the tourmaline group related to the end-member dravite, ideally NaMg3Al6Si6O18(BO3)3(OH)3(OH), by the substitution F → (OH) (Clark et al., 2011).
In order to assess the stability of the primary alluaudite - triphylite assemblage, hydrothermal experiments were performed between 400 and 800°C, starting from the LiNa2MnxFe3-x 2+Fe3+(PO4)4 compositions (x = 1.054, 1.502, 1.745) that represent the ideal compositions of the alluaudite + triphylite assemblages from the Kibingo (Rwanda), Hagendorf-Süd (Germany), and Buranga (Rwanda) pegmatites, respectively. Secondary ion mass spectrometry was used at our knowledge for the first time to measure Li in all the Li-bearing phosphates over a wide concentration range spanning from few ppm Li up to ~11 wt% Li2O. Our SIMS analyses of the synthesized phosphates indicate that the Li contents of alluaudites, maricites, and X-phase increase progressively with temperature, while the Li content of triphylite-type phosphates decreases due to the Li → Na substitution. The Na-exchange equilibrium between triphylite-type phosphates and alluaudite is correlated with the temperature according to the equation:
ln(xNaTri/xNaAll) = -7.0(7) 103/T + 5.4(9)
This equation can be used to estimate the crystallization temperature of triphylite–alluaudite assemblages independently of the oxygen fugacity (Hatert et al., 2011).
Fig. 22- Temperature dependence of the Li/(Li+Na) ratios, for the phosphates synthesized in the LiNa2MnxFe3-x2+Fe3+(PO4)4 (x = 1.054, 1.502, 1.745) system. Circles = alluaudites, triangles = triphylite, squares = X-phase, crosses = maricite (Hatert et al., 2011). |
A Ba-Ti rich oxymica occurs in an olivine nephelinite from S. Demetrio High in the northern margin of the Hyblean Plateau (Sicily, Italy). The studied oxykinoshitalite, characterized by micro-Raman spectroscopy, electron microprobe WDS (wavelength dispersive system), and secondary-ion mass spectrometry (SIMS), was compared with the type material from the Fernando de Noronha island (Pernambuco, Brazil). Structural formula of the Hyblean oxykinoshitalite, calculated on the basis of 7 (Si, Al, Fe, Mg, Ti), is (Ba0.51K0.41Na0.04Ca0.01)S0.97(Mg1.98Fe0.55Ti0.48)S3.01(Si2.42Al1.56)S3.98 O10[O1.17F0.62(OH)0.21]S2.00.
The lack of chemical zoning and the enrichment in Zr and Nb in the groundmass crystals of the Hyblean oxykinoshitalite suggest formation during the final crystallization stage of a basaltoid magma with ocean island basalt (OIB) affinity. Most likely, the magma originated by partial melting of metasomatized ultramafic rocks in the Hyblean crustal basement (Manuella et al., 2012).
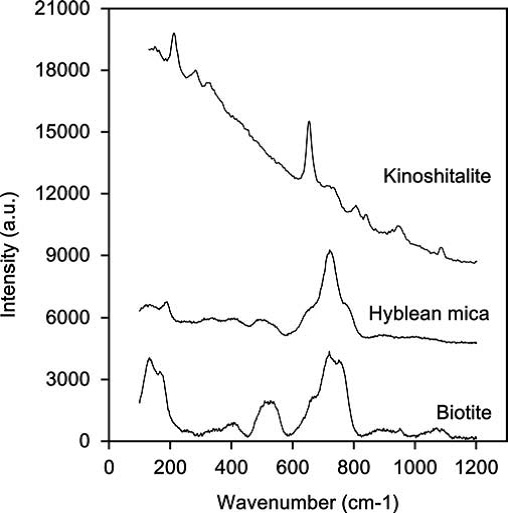
Fig. 23- Raman spectra of three trioctahedral micas (i.e., Hyblean mica, kinoshitalite and biotite), in the range 1200–100 cm_1 (see Manuella et al., 2012 for details). |
The contribution of Secondary Ion Mass Spectrometry (SIMS) -with particular reference to the experience acquired over these last years at the (Cameca IMS 4f) ion probe lab. of CNR-IGG, Pavia (Italy)- to the study of crystal chemistry of mica minerals was described in the Chapter 43 by Ottolini et al. (2012) in the Mass Spectrometry Handbook (see below).
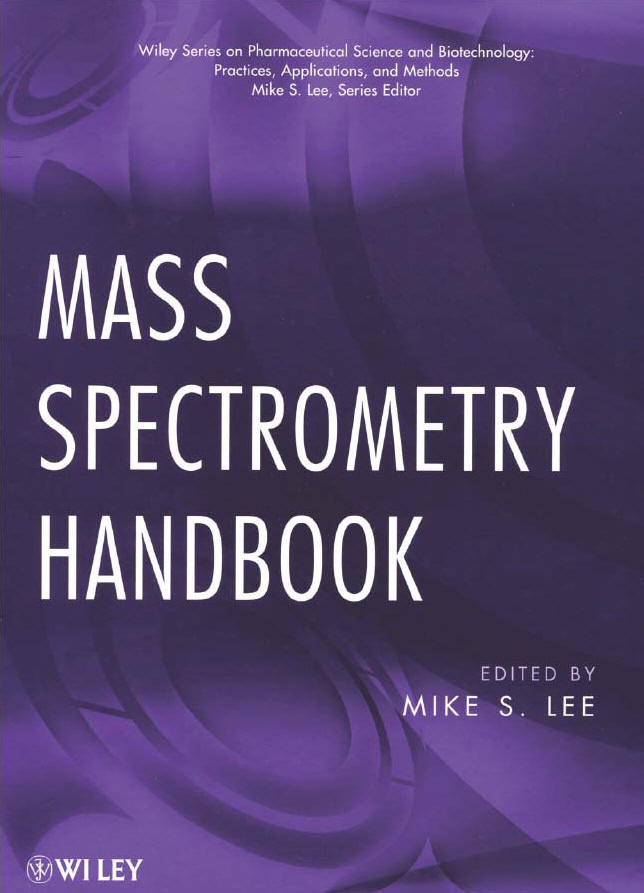
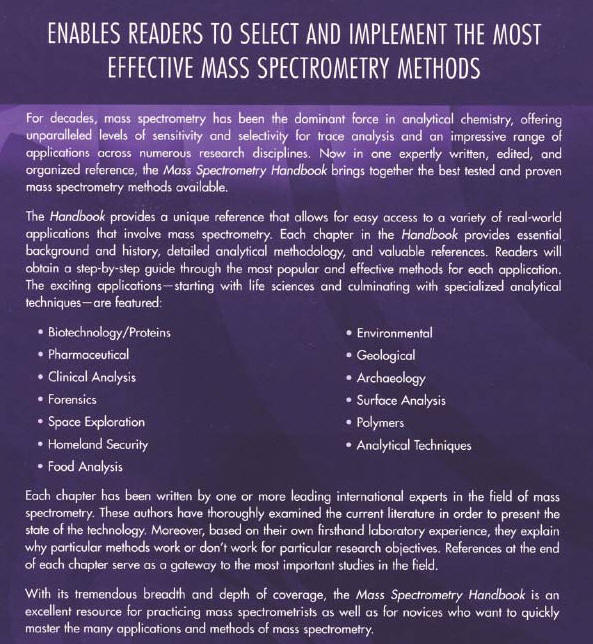
The beryl crystals studied by Aurisicchio et al.(2012) are found in miarolitic pockets of pegmatitic dikes outcropping in the southeastern sector of the Monte Capanne pluton, on the Island of Elba, Italy. We report the chemical composition of the beryl crystals, and in particular the measurement of their content of light elements carried out using the EMPA and SIMS techniques. In addition to previous techniques, BSE images and X-ray maps have been recorded. Boron was detected together with Li and Be at selected spots. Our results for B represent the first direct measurements available for this element in the structure of beryl. The above analytical methods allowed us to acquire complete knowledge of each crystal in terms of in situ elemental concentration and their spatial distribution.The outcome of complex light element substitutions at very small scale (on the order of a few micrometers) emphasizes the importance of SIMS for the analysis of silicates for the light elements.
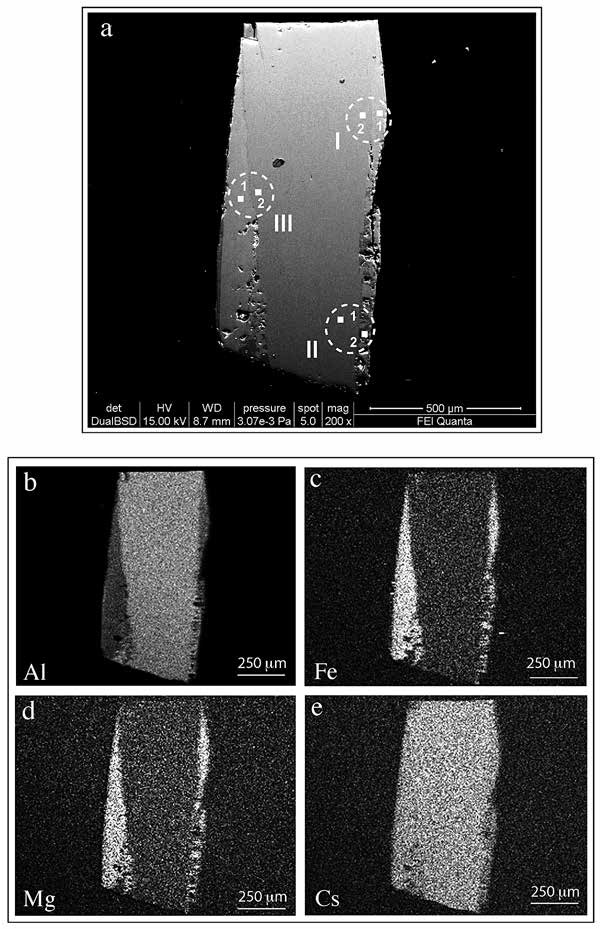
Fig. 24- Back-scattered electron image of sample 43, showing two pale gray side-bands bordering a volumetrically dominant gray zone with lower contrast (a). The X-ray maps show the distribution of Al (b), Fe (c), Mg (d) and Cs (e) through the crystal. The squares shown on the BSE image represent the positions of EMPA and SIMS spots (see Aurisicchio et al., 2012 for details). |
Iron-manganese phosphates are common accessory minerals occurring in granitic pegmatites, in metamorphic rocks, and in meteorites. In rare-elements pegmatites, primary phosphates of the triphylite-lithiophilite series [Li(Fe2+,Mn2+)(PO4)-Li(Mn2+,Fe2+)(PO4)] form masses that can reach several meters in diameter, enclosed in silicates. During the oxidation processes affecting the pegmatites, these olivine-type phosphates progressively transform to ferrisicklerite-sicklerite [Li1-x(Fe3+,Mn2+)(PO4)-Li1-x(Mn2+,Fe3+)(PO4)] and to heterosite-purpurite [(Fe3+,Mn3+)(PO4)-(Mn3+,Fe3+)(PO4)], according to the substitution mechanism Li+ + Fe2+ → ☐ + Fe3+. In order to shed some light on the structural modifications induced by the oxidation processes affecting lithiophilite, we decided to investigate a natural sample from the Altai Mountains, China, in which a progressive transition from lithiophilite to sicklerite is observed. The results of the chemical and structural characterizations of this exceptional sample are given in the paper by Hatert et al. (2012).
The topotactic oxidation and delithiation reaction from triphylite, Li(Fe,Mn)PO4, leading to ferrisicklerite, Li<1(Fe3+,Mn2+)PO4, was investigated under hydrothermal conditions. At 120 °C a rim of 0.1 mm thickness of ferrisicklerite had formed around the core of unreacted triphylite. The sharp reaction boundary was clearly visible, due to the reddish brown absorption colors of ferrisicklerite, compared to colorless triphylite. Using single-crystal X-ray diffraction (XRD), secondary ion mass spectrometry (SIMS), electron probe micro-analysis (EPMA) and 57Fe-Mössbauer spectroscopy the product ferrisicklerite was characterized and its composition determined as Li0.30(7)(Fe2+ 0.049(1)Fe3+0.65(2)Mn2+ 0.218 (5)Mg0.062(2))0.98(1)P1.01(3)O4. EPMA investigations across the reaction boundary showed no changes in the concentrations of Fe, Mn, Mg, and P. In contrast, SIMS measurements clearly proved the delithiated state of the ferrisicklerite product. Our experimental results demonstrate that the existence of primary ferrisicklerite in granitic pegmatites, reported in literature, could be better interpreted as an oxidation and replacement product of triphylite, formed under low-temperature and highly oxidizing hydrothermal conditions (Schmid-Beurmann et al., 2013).
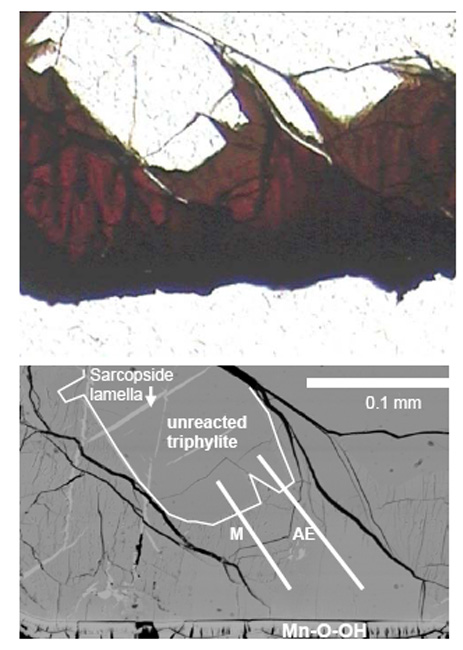
Fig. 25- BSE (down) and transmitted light optical micrographs (up) within the rectangular areas with the transects M and AE (bold white line) investigated by SIMS (5 µm Ø 16O– primary ion beam), and reaction front between triphylite and ferrisicklerite (thin white line). See Schmid-Beurmann et al. (2013), for details. |
The new mineral strontiohurlbutite, a Sr-dominant analog of hurlbutite, was discovered in the Nanping No. 31 pegmatite, Fujian province, southeastern China. The species and the name have been approved by the International Mineralogical Association, Commission on New Minerals, Nomenclature and Classification (CNMNC) (IMA 2012-032) (Williams et al. 2012).
The BeO content of strontiohurlbutite was determined by accurate SIMS analysis at CNR-IGG, Pavia. The calibration factor for Be in the beryllonite standard (courtesy of S. Philippo, Natural History Museum of Luxembourg) was obtained through the calculation of the experimental Be ion yield, having chosen P as the inner element for the matrix. We thus derived the IY(Be/P), defined as (Be+/P+)/[(Be(at)/P(at)] where Be+ and P+ are the current intensities detected at the electron multiplier and (at) is the elemental atomic concentration. The IY (Be/P) was then used to calculate the Be concentration in the strontiohurlbutite, resulting in 17.71 ± 0.464(1s) BeO wt% (ideal value: BeO = 16.92 wt%). Additionally, H2O content by 0.066 ± 0.010(1s) wt% was quantified in strontiohurlbutite; lithium is absent (Rao et al., 2014).
Muscovite, KAl2[AlSi3O10](OH)2, is a common rock-forming mineral in igneous and metamorphic-rocks, sediments, hydrothermal alteration and ore deposits. The site between two adjacent T-O-T (tetrahedral-octahedral-tetrahedral) layers is shared between K and NH4 in all proportions leading to the building of the “ammonium micas”. In Ottolini et al. (2014) three tobelite crystals were investigated in terms of major and minor constituents by electron probe microanalysis (EPMA) and in terms of nitrogen, for the first time at authors’ knowledge, by secondary ion mass spectrometry (SIMS). The objective was that to gain information on the presence and amount of NH4 in this matrix. The lack of standards did not allow us to provide quantitative results at the ion microprobe. Nevertheless, the SIMS data agree qualitatively with constraints resulting from EPM analyses and charge-balance crystal chemical considerations.
The results of this study emphasize the capabilities of SIMS in the in-situ analysis of N and point out the possibility to develop reference materials (standards) for quantitative SIMS analysis of nitrogen in mica.
Fig. 26- Representative mass scans of peaks of interest at mass 14, obtained by Cameca IMS 4f ion microprobe at a mass resolving power of 1250 (M/ΔM) for the samples analysed: Tob_m2, Tob_m3, and Tob_3. The resolved peaks (from next to right) are: 28Si2+, 14N+ and 12CH2+ (see Ottolini et al. 2014, for details) |
Piston-cylinder experiments were performed by (Laporte et al. 2014) to characterize the composition of liquids formed at very low degrees of melting of two fertile lherzolite compositions with 430 ppm and 910 ppm K2O at 1 and 1.3 GPa. This study brings a strong support to the hypothesis that phonolitic lavas or their plutonic equivalents (nepheline syenites) may be produced directly by partial melting of upper mantle rock-types at moderate pressures (1–1.5 GPa), especially where large domains of the subcontinental lithospheric mantle has been enriched in potassium by metasomatism. The circulation of low-degree partial melts of peridotites into the upper mantle may be responsible for a special kind of metasomatism characterized by Si- and alkali-enrichment. When they are unable to escape by porous flow, low-degree melts will ultimately be trapped inside neighboring olivine grains and give rise to the silica- and alkali-rich glass inclusions found in peridotite xenoliths.
A drawback of the experiments at low degrees of melting is that any trace of water in the capsule will partition preferentially into the partial melt, yielding significant amounts of dissolved water even under nominally anhydrous conditions. Dissolved water contents in some experimental glasses were measured using our ion microprobe Cameca IMS 4f with a beam diameter of 5 µm. The water contents are relatively low and decrease with increasing melt fraction F : from 1.1 wt.% H2O at our lowest degrees of melting (F ~ 1 wt.%) to 0.1 wt.% H2O at F = 12.7 wt.%.
The relationship between water content of experimental glasses and melt fraction F (both in wt.%) for our whole data set (9 partial melting experiments, including three from Laporte et al., 2004) can be described by a single law:
H2O=1.350 e0.176 F
with a coefficient of determination equal to 0.983. Further information in Laporte et al. (2014).
Amphiboles are the most widespread hydrous metasomatic phases in spinel-bearing mantle peridotites from Harrow Peaks (HP), Northern Victoria Land (Antarctica). They occur both in veinlets and disseminated in the peridotite matrix (preferentially associated with clinopyroxene and spinel grains). Four amphibole crystals were investigated by single-crystal X-ray diffraction (SC-XRD), electron microprobe analysis (EMPA), secondary ion mass spectrometry (SIMS) and micro-Mössbauer spectroscopy (Gentili et al., 2015). The crystalchemical data allowed to constrain upper mantle conditions during growth of these amphiboles and the role of volatile circulation during metasomatic processes in the Antarctic region. The W-site is mainly occupied by O2- (0.984-1.187 apfu) plus OH (H2O: 0.70–1.01 wt%) and minor F (0.04–0.24 wt%) and Cl (0.03–0.08 wt%). Consequently, HP amphiboles are actually characterized by a significant oxo component. The low volatile contents, in particular OH, in the amphibole, are accompanied by records of high aH2O (up to 0.8, close to the water saturation) and fO2. This unusual feature with respect to mantle amphiboles from various tectonic settings worldwide, and in particular to amphiboles from Baker Rocks (BR, Perinelli et al., 2012; Bonadiman et al., 2014), suggests that this mantle region records different physico-chemical conditions (i.e., T, redox conditions and melt H2O contents). For further info, see the paper (Gentili et al., 2015).
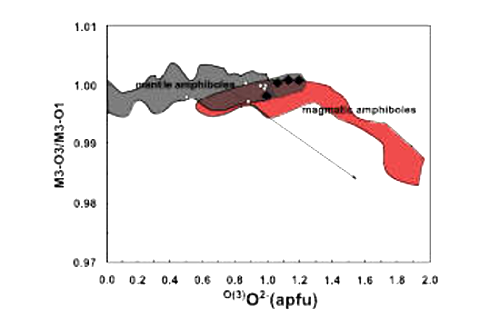
Fig. 27- M(3)–O3/M(3)–O1 versus O(3)O2- (degree of dehydrogenation) (Gentili et al., 2015). Filled diamonds: Harrow Peaks amphiboles (data from SIMS measurements). Open diamonds: BR amphiboles (Bonadiman et al., 2014). |
In the study by Schingaro et al., (2016), hydrogen, fluorine, and lithium were measured in a suite of Ti-garnets from rock types of different origin and provenance, by means of secondary ion mass spectrometry (SIMS) at CNR-IGG, Pv. So far, this technique was only used to derive an H2O calibration curve employing, however, garnets with pyralspite composition, whose hydrogen abundance was determined by manometry and IR measurements (Koga et al., 2003). Multiple mechanisms have been proposed to describe the hydrogen uptake in garnets. The hydrogarnet substitution (4H + Z☐ → ☐+ ZSi), where, i.e., a SiO4 unit may be replaced by H4O4 on the tetrahedral site, was often invoked because consistent with diffraction technique data. Fluorine content of Ti-andradites or titanium andradites-grossular was mainly obtained by electron-microprobe analysis and by F-sensitive glass electrode. Exchange reaction F- → OH- was used to explain the incorporation of fluorine in garnet, but also more complex reactions were proposed involving coupled cations substitutions for charge balance. To the best of our knowledge, studies on lithium in Ti-garnets, instead, are missing in literature. The results of SIMS, electron microprobe analysis (EPMA), X-ray powder diffraction (XRPD), single-crystal X-ray diffraction (SCXRD), and Mössbauer spectroscopy have been integrated to provide a comprehensive crystal chemical characterization of the studied samples (see Schingaro et al. 2016 for details).
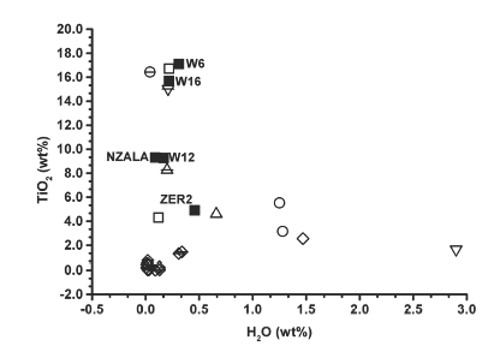
Fig. 28- Plot of the TiO2 (wt%) vs. measured H2O (wt%) in Ti-garnets (Schingaro et al., 2016). Symbols: solid squares = samples of this work; open symbols = samples from literature; circle = 80802 and 80303 from Onuki et al. (1982); pointing downward triangle = SB-3 from Lager et al. (1989); pointing upward triangle = SA12 from Müntener and Hermann (1994); diamond = 31/B from Ulrych et al. (1994); circle with horizontal line = Ice River crystal from Locock et al. (1995); pointing downward triangle with horizontal line = AF-05 from Chakhmouradian and McCammon (2005); pointing upward triangle with horizontal line = M-1 from Katerinopoulou et al. (2009); diamond with horizontal line = KPK39-1-1, KPK54-10, KPK54-11, KTK05, KTK07, KTK09, KTK10, KPK56-12-2, KPK56-12-9, KPN09, KPN10, and KPN11 from Phichaikamjornwut et al. (2011). |
Sodalite-group minerals (SGM) commonly occur in alkaline igneous rocks, and in some hydrothermal and metamorphic rocks. Their structural cavities are usually occupied by Cl-, SO42-, CO32-, S2-, S3-, OH-, H2O, CO2. Cationic and anionic contents of SGM are of interest for the study of magmatic systems and in different fields of material science, since their (ultra)microporous structures show high flexibility and versatility in hosting tetrahedral cations and variable channels/cavities components. In the paper by Balassone et al., 2016 (in press), we have investigated the volatile contents in a set of samples SGM, associated with historical lavas of the Vesuvius. The main results are summarized in the following:
SIMS: this is the first SIMS study on quantitative measurements of H, C and F in SGM. The higher H2O and CO2 amounts and the larger variations have been detected in S-sodalite. F does always occur in very low amounts. The inverse correlation observed for S and C in S-sodalite and haüyne can be justified by the SO42- - CO32 substitution in their extra-framework X site.
µ-FTIR: this technique has pointed out homogeneous vs. heterogeneous distribution of C and H (the latter more frequently). In some cases high values can be due to the presence of micro-fractures and/or grain boundaries that are more affected by fluid action, and/or to the presence of fluid inclusions in the crystal.
GEOLOGICAL IMPLICATIONS: some of the lower contents of C and H are found in the SGM samples, i.e. S-sodalite E5707 and haüyne 22123 (respectively related to AD 1872 and AD 1906 eruptions). We speculate that SGM from samples related to historical lavas were trapped in the uprising magma and pervaded by fluids escaping from the melt. In accepting this speculative hypothesis, the H2O and CO2 contents of sodalite from xenoliths are dependent on the composition and decompression history of hosting magma, thus providing additional information on the volcanic processes.
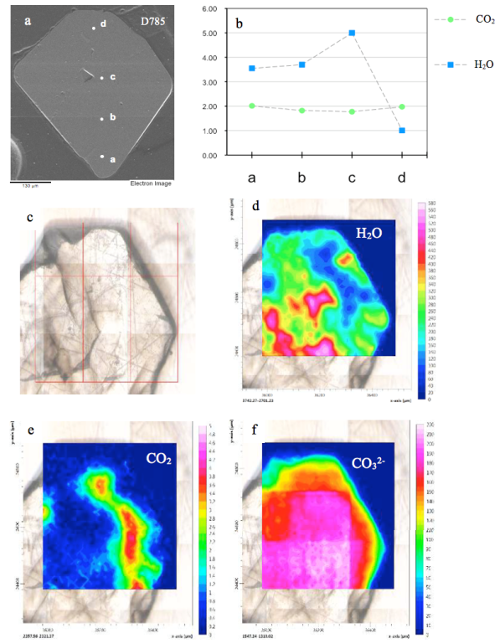
Fig. 29- A representative SGM sample with a heterogeneous H and C distribution. The analyses were carried out on two fragments coming from the same sample, i.e. sulphatic sodalite D785. (a) BSE micrograph, showing the single SIMS spots location; (b) the corresponding SIMS H2O and CO2 amounts in wt.%. The four SIMS spots across the sample show a strong H2O zonation, with up to 5 wt.% water in the core (SIMS spot c), also confirmed by µ-FTIR FPA data. As observed in transmitted light microphotograph (c), this feature could be also due to micro-fractures occurring throughout the crystal; (e) the CO2 contents seem to be inversely related to H2O concentrations (d), whereas (f) the CO32- distribution seems to be more homogeneous (see Balassone et al., 2016, for details). |
References cited
Ottolini L., Bottazzi P., Vannucci R.: Quantification of Lithium, Beryllium and Boron in Silicates by Secondary Ion Mass Spectrometry Using Conventional Energy Filtering, Anal. Chem., 65, (1993), 1960-1968.
Hawthorne F.C., Cooper M.A., Bottazzi P., Ottolini L., Scott Ercit T., Grew E.S.: Micro-analysis of minerals for boron by SREF, SIMS and EMPA: A comparative study, Canad. Mineral., 33, (1995a), 389-397.
Ottolini L., Bottazzi P., Zanetti A.: Quantitative Analysis of Hydrogen, Fluorine and Chlorine in Silicates Using Energy Filtering, SIMS IX, Proceedings of the 9th International Conference on Secondary Ion Mass Spectrometry, Yokohama (Japan), Nov. 7-12 (1993), A. Benninghoven, Y. Nihei, R. Shimizu and H.W. Werner (Eds.), John Wiley & Sons, Chichester (England), (1994), 191-194.
Ottolini L., Camara F., Bigi S.: An investigation of matrix effects in the analysis of fluorine in humite-group minerals by EMPA, SIMS and SREF, Amer. Mineral., 85, (2000), 89-102.
Camara F. & Ottolini L.: New data on the crystal-chemistry of fluoborite by means of SREF, SIMS and EMP analysis, Amer. Mineral., 85, (2000), 103-107.
Ottolini L. & Camara F.: An investigation of matrix effects in the analysis of fluorine in humite-group minerals, SIMS XII, Proceedings of the 12th International Conference on Secondary Ion Mass Spectrometry, Brussels (Belgium), Sept. 5-10 (1999), A. Benninghoven, P. Bertrand, H.-N. Migeon and H.W. Werner (Eds.), Elsevier Science B.V., Amsterdam (The Netherlands), (2000), 365-368.
Tribuzio R., Riccardi M.P., Ottolini L.: Trace element redistribution in high- temperature deformed gabbros from East Ligurian ophiolites (Northern Apennines, Italy): constraints on the origin of syndeformation fluids, J. metam. Geol., 13, (1995), 367-377.
Ottolini L., Bottazzi P., Zanetti A., Vannucci R.: Determination of Hydrogen in Silicates by Secondary Ion Mass Spectrometry, The Analyst, 120, (1995), 1309-1313.
Alberico A.& Ottolini L.: Quantification of H2O content in carlosturanite: comparison of SIMS, TGA and EMPA data, GEOITALIA 1997, Bellaria (Rimini), Oct. 5-9 (1997), Plinius, 18, 25-26.
Ottolini L. & Hawthorne F.C.: SIMS ionization of hydrogen in silicates: a case study of kornerupine, J. Anal. Atom. Spectrom., 16,(2001), 1266-1270.
Ottolini L. & Camara F.: SIMS Analysis of H in chemically-complex silicate matrixes, SIMS XIII, 13th Int. Conference on Secondary Ion Mass Spectrometry, Nov. 11-16 (2001), Nara (Japan), Abstracts Volume, 226.
Ottolini L., Camara F., Hawthorne F.C.: Quantification of H, B, F in kornerupine: Accuracy of SIMS and SREF (X-Ray Single-Crystal Structure-Refinement) Data, Mikrochim. Acta, 139, (2002), 125-129.
Braga R., Callegari A., Messiga B., Ottolini L., Renna M.R., Tribuzio R.: Origin of prismatine from the Sondalo granulites (Central Alps, Northern Italy), Eur. J. Mineral., dedicated to the memory of Luciano Ungaretti, 15, (2003), 393-400.
Ottolini L. & Hawthorne F.C.: An investigation of SIMS matrix effects on H, Li and B ionization in tourmaline, Eur. J. Mineral., 11, (1999), 679-690.
Selway J.B., Novák M., Hawthorne F.C., Cerný P., Ottolini L., Kurtis Kyser T.: Rossmanite, ¨ (LiAl2)Al6(Si6O18)(BO3)3(OH)4, a new alkali-deficient tourmaline: Description and crystal structure, Amer. Mineral., 83, (1998), 896-900.
Aurisicchio C., Demartin F., Ottolini L., Pezzotta F.: Homogeneous liddicoatite from Madagascar: a possible reference material? First EMPA, SIMS and SREF data, Eur. J. Mineral., 11, (1999a), 237-242.
Aurisicchio C., Ottolini L., Pezzotta F.: Electron- and ion-microprobe analyses, and genetic inferences of tourmalines of the foitite-schorl solid solution, Elba Island (Italy), Eur. J. Mineral., 11, (1999b), 217-225.
Teertstra D.K., Cerný P., Ottolini L.: Stranger in paradise: liddicoatite from the High Grade Dike pegmatite, southeastern Manitoba, Canada, Eur. J. Mineral., 11, (1999), 227-235.
Novák M., Selway J.B., Cerný P., Hawthorne F.C., Ottolini L.: Tourmaline of the elbaite-dravite series from an elbaite-subtype pegmatite at Bližná, southern Bohemia, Czech Republic, Eur. J. Mineral., 11, (1999a), 557-568.
Camara F., Ottolini L., Hawthorne F.C.: Crystal-chemistry of three tourmalines by SREF, EMPA and SIMS, Amer. Mineral., 87, (2002), 1437-1442.
Andreozzi G.B., Ottolini L., Lucchesi S., Graziani G., Russo U.: Crystal chemistry of the axinite-group minerals: A multi-analytical approach, Amer. Mineral., 85, (2000), 698-706.
Malcherek T., Domeneghetti M.C., Tazzoli V., Ottolini L., McCammon C., Carpenter M.A.: Structural properties of ferromagnesian cordierites, Amer. Mineral., 86, (2001), 66-79.
Cerný P., Novák M., Margison S., Selway J.B., Ottolini L., Brown J.: Boron in rare-element granitic pegmatites: tourmaline, borates, micas and feldspars, IMA2002, 18th General Meeting of the International Mineralogical Association, Edinburgh, Sept. 1-6, (2002), Abstract, 205.
Cerný P., Chapman R., Schreyer W., Ottolini L., Bottazzi P., McCammon C.: Lithium in sekaninaite from the type locality, Dolní Bory, Czech Republic, Canad. Mineral., 35, (1997), 167-173
Hawthorne F.C., Ungaretti L., Oberti R., Caucia F., Callegari A.: The crystal-chemistry of staurolites. I: Crystal structure and site populations. Canad. Mineral., 31, (1993), 551-582.
Oberti R., Hawthorne F.C., Zanetti A., Ottolini L., Soto J.I.: Crystal-structure refinement of a highly ordered staurolite, Canad. Mineral., 34, (1996), 1051-1057.
Oberti R., Ungaretti L., Tlili A., Smith D.C., Robert J.-L.: The crystal structure of preiswerkite, Amer. Mineral., 78, (1993), 1290-1298.
Liang J.-J., Hawthorne F.C., Novák M., Cerný P., Ottolini L.: Crystal-structure refinement of boromuscovite polytypes using a coupled Rietveld - Static-Structure Energy-Minimization Method, Canad. Mineral., 33, (1995), 859-865.
Cerný P., Stanek J., Novák M., Baadsgaard H., Rieder M., Ottolini L., Kavalová M., Chapman R.: Geochemical and structural evolution of micas in the Rozná and Dobrá Voda pegmatites, Czech. Republic, Mineral. Petrol., 55, (1995), 177-201.
Novák M., Cerný P., Cooper M.A., Hawthorne F.C., Ottolini L., Xu Z., Liang J.-J.: Boron-bearing 2M1 polylithionite and 2M1 + 1Mboromuscovite from an elbaite pegmatite at Recice, western Moravia, Czech Republic, Eur. J. Mineral., 11, (1999b), 669-678.
Nimis P., Molin G., Visonà D.: Crystal chemistry of danalite from Daba Shabeli Complex (Somalia), Mineral. Mag., 60, (1996), 375-379.
Hawthorne F.C., Ungaretti L., Oberti R., Bottazzi P., Czamanske G.K.: Li: an important component in igneous alkali amphiboles. Amer. Mineral., 78, (1993), 733-745.
Zanetti A., Sardone N., Oberti R, Vannucci R., Ottolini L., Bottazzi P.: Crystal-chemical changes in amphiboles from Zabargad peridotite: the reconstruction of a complex sub-solidus evolution. IMA 16th General Meeting. Pisa, 4-9 Settembre, (1994), Abstracts Volume, 457.
Hawthorne F.C., Ungaretti L., Oberti R., Cannillo E., Smelik E.A.: The mechanism of [6]Li incorporation in amphibole. Amer. Mineral., 79, (1994), 443-451.
Hawthorne F.C., Oberti R., Cannillo E., Sardone N., Zanetti A., Grice J.D., Ashley P.M.: A new anhydrous amphibole from Hoskins mine, Grenfell, New South Wales, Australia: Description and crystal structure of ungarettiite, NaNa2(Mn22+Mn33+)Si8O22O2. Amer. Mineral., 80, (1995b), 165-172.
Hawthorne F.C., Oberti R., Ottolini L., Foord E.E.: Lithium-bearing fluor-arfvedsonite from Hurricane Mountain, New Hampshire: A crystal-chemical study, Canad. Mineral., 34, (1996a), 1015-1019.
Hawthorne F.C., Oberti R., Ungaretti L., Ottolini L., Grice J.D., Czamanske G.K.: Fluor-ferro-leakeite, NaNa2(Fe22+Fe23+Li)Si8O22F2, a new alkali amphibole from the Canada Pinabete pluton, Questa, New Mexico, U.S.A., Amer. Mineral., 81, (1996b), 226-228.
Zanetti A., Oberti R., Bottazzi P., Vannucci R.: Fe3+ contents in partially-dehydrogenated high-T amphiboles by combining SREF, EMP and SIMS analyses: an insight into intensive parameters of upper mantle. EUG 9, Strasbourg, France, 23-27 March, (1997), Terra Nova, 9, 437.
Hawthorne F.C., Oberti R., Zanetti A., Czamanske G.K.: The role of Ti in hydrogen-deficient amphiboles: sodic-calcic and sodic amphiboles from Coyote Peak, California, Canad. Mineral., 60, (1998), 925-935.
Tiepolo M., Zanetti A., Oberti R.: Detection, crystal-chemical mechanisms and petrological implications of [6]Ti4+ partitioning in pargasite and kaersutite, Eur. J. Mineral., 11, (1999), 345-354.
Zanetti A., Oberti R., Piccardo G.B., Vannucci R.: Light lithophile (Li, Be and B), volatile (H, F and Cl) and trace elements composition of mantle amphiboles from Zabargad peridotite: insights into the multistage subsolidus evolution of subcontinental mantle during Red Sea rifting, Goldschmidt 2000, September 3rd-8th, (2000), Oxford (UK), J. Conf. Abstracts, 5(2), 1120.
Hawthorne F.C., Cooper M.A., Grice J.D., Ottolini L.: A new anhydrous amphibole from the Eifel region, Germany: Description and crystal structure of obertiite, NaNa2(Mg3Fe3+Ti4+)Si8O22O2, Amer. Mineral., 85, (2000), 236-241.
Oberti R., Caballero J.M., Ottolini L., López-Andrés S., Herreros V.: Sodic-ferripedrizite, a new monoclinic amphibole bridging the magnesium-iron-manganese-lithium and the sodium-calcium groups, Amer. Mineral., 85, (2000), 578-585.
Hawthorne F.C., Oberti R., Cannillo E., Ottolini L., Roelofsen J., Martin R.M.: Li-bearing arfvedsonitic amphiboles from the Strange Lake peralkaline granite, Quebec, Canad. Mineral., 39, (2001), 1161-1170.
Caballero J.M., Oberti R., Ottolini L.: Ferripedrizite, a new monoclinic BLi amphibole end-member from the Eastern Pedriza Massif, Sierra de Guadarrama, Spain, and a restatement of the nomenclature of Mg-Fe-Mn-Li amphiboles, Amer. Mineral., 87, (2002), 976-982.
Oberti R., Camara F., Ottolini L., Caballero J.M.: Lithium in amphiboles: detection, quantification, crystal-chemical mechanisms and definition of a compositional space bridging sodic and BLi-amphiboles, Eur. J. Mineral., dedicated to the memory of Luciano Ungaretti, 15, (2003a), 309-319.
Oberti R., Camara F., Caballero J.M., Ottolini L.: Sodic-ferri-ferropedrizite and ferri-clinoferroholmquistite, mineral data and degree of order of the A-site cations in Li-rich amphiboles, Canad. Mineral., 41, (2003b), 1345-1354.
Oberti R., Camara F., Caballero J.M.: Ferri-ottoliniite and ferriwhittakerite, two new end-members of the new Group 5 for monoclinic amphiboles, Amer. Mineral., 89, (2004), 888-893.
Tait K.T., Hawthorne F.C., Grice J.D., Ottolini L., Nayak V.K : Dellaventuraite, NaNa2(MgMn3+2Ti4+Li)Si8O22O2, a new anhydrous amphibole from the Kajlidongri Manganese Mine, Jhabua District, Madhya Pradesh, India. Amer. Mineral., 90, (2005), 304-309.
Oberti R., Cámara F., Ottolini L.: Clinoholmquistite discredited: the new amphibole end-member fluoro-sodic-pedrizite, Amer. Mineral., 90, (2005), 732-736.
Raade G., Bernhard F., Ottolini L.: Replacement textures involving four scandium silicate minerals in the Heftetjern granitic pegmatite, Norway, Eur. J. Mineral., 16, (2004), 945-950.
Andreozzi G.B., Ottolini L., Lucchesi S., Graziani G., Russo U.: Crystal chemistry of the axinite-group minerals: A multi-analytical approach, Amer. Mineral., 85, (2000), 698-706.
Ottolini L. & McDonough W.F.: Geochemistry of Lithium and Boron in the Mantle: Results from Studies of Peridotites, Goldschmidt Conference, Heidelberg, March 30-April 4 (1996), J. Conf. Abstracts, Cambridge Publications, 1, 446.
Ottolini L., Le Fevre B., Vannucci R.: Direct assessment of mantle boron and lithium contents and distribution by SIMS analyses of peridotite minerals, Earth Planet. Sci. Lett., 228, (2004), 19-36.
Scambelluri M., Müntener O., Ottolini L., Pettke T., Vannucci R.: Boron and chlorine cycling in the subducted hydrous oceanic mantle, Goldschmidt Conference, Davos, Aug. 18-23 (2002), Goldschmidt Conf. Abstracts 2002, Pergamon, A671.
Scambelluri M., Müntener O., Ottolini L., Pettke T.T., Vannucci R.: The fate of B, Cl and Li in the subducted oceanic mantle and in the antigorite breakdown fluids, Earth Planet. Sci. Lett., 222, (2004), 217-234.
Wulff-Pedersen E., Neumann E.-R., Vannucci R., Bottazzi P., Ottolini L.: Silicic melts produced by reaction between peridotite and infiltrating basaltic melts: ion probe data on glasses and minerals in veined xenoliths from La Palma, Canary Islands, Contrib. Mineral. Petrol., 137, (1999), 59-82.
Freda C., Baker D.R., Ottolini L.: Reduction of water loss from gold-palladium capsules during piston-cylinder experiments by use of pyrophyllite powder, Amer. Mineral., 86, (2001), 234-237.
Baker D.R., Conte A.M., Freda C., Ottolini L.: The effect of halogens on Zr diffusion and zircon dissolution in hydrous metaluminous granitic melts, Contrib. Mineral. Petrol., 142, (2002), 666-678.
Ottolini L., Camara F., Hawthorne F.C., Stirling J.: SIMS matrix effects in the analysis of light elements in silicate minerals: Comparison with SREF and EMPA data, Amer. Mineral., 87, (2002), 1477-1485.Bellatreccia F., Della Ventura G., Ottolini L., Libowitzky E., Beran A.: The quantitative analysis of OH in vesuvianite: a polarized FTIR and SIMS study, Phys. Chem. Miner., 32, (2005a), 65-76.
Kägi R., Müntener O., Ulmer P., Ottolini L.: Piston-cylinder experiments on H2O undersaturated Fe-bearing systems: An experimental setup approaching fO2 conditions of natural calc-alkaline magmas, Amer. Mineral., 90, (2005), 708-717.
Kogarko L.N., Uvarova Y.A., Sokolova E., Hawthorne F.C., Ottolini L., Grice J.D.: Oxykinoshitalite, a new species of mica from Fernando De Norohna Island, Pernambuco, Brazil: occurrence and crystal structure, Canad. Mineral., 43, (2005), 1501-1510.
Mesto E., Schingaro E., Scordari F., Ottolini L.: An electron microprobe analysis, secondary ion mass spectrometry and single crystal X-ray diffraction study of phlogopites from Mt. Vulture, Potenza, Italy: Consideration of cation partitioning, Amer. Mineral., 91,(2006), 182-190.
Médard E., Schmidt M.W., Schiano P., Ottolini L.: Melting of Amphibole-bearing Wehrlites: An Experimental Study on the Origin of Ultra-calcic Nepheline-normative Melts, J. Petrol., 47, (2006), 481-504.
Ottolini L., Cámara F., Hawthorne F.C.: Strategies for Quantification of Light Elements in Minerals by SIMS: H, B and F, Microchim. Acta, 155, (2006), 229-233.
Le Fèvre B., Ottolini L.: Preparation of Reference Glasses for in-situ Analysis of Lithium and Boron, Microchim. Acta, 155, (2006), 189-194.
Bellatreccia F., Ottolini L., Della Ventura G.: Microanalysis of Hydrogen, Boron and Fluorine in Vesuvianite by Means of SIMS, EPMA and FTIR, Microchim. Acta, 155, (2006), 91-94.
Raffone N., Le Fèvre B., Ottolini L., Vannucci R., Zanetti A.: Light-Lithophile Element Metasomatism of Finero Peridotite (W ALPS): A Secondary-Ion Mass Spectrometry Study, Microchim. Acta, 155, (2006), 251-255.
Aurisicchio C., Ottolini L., De Vito C. :Tracing the archaeological emerald origin by EMPA and SIMS data, 30th Intern. Gemmological Conference (IGC 2007), Moscow, Russia 15-19 July, 2007, Abstracts Volume, pages 8-9 (with Appendix 1).
Gianfagna A., Scordari F., Mazziotti-Tagliani S., Ventruti G., Ottolini L.: Fluorophlogopite from Biancavilla (Mt Etna, Sicily, Italy): crystal structure and crystal chemistry of a new F-dominant analogue of phlogopite, Amer. Mineral., 92, (2007), 1601-1609.
Scordari F., Schingaro E., Ventruti G., Lacalamita M., Ottolini L.: Red micas from basal ignimbrites of Mt. Vulture (Italy): interlayer content appraisal by a multi-methodic approach, Phys. Chem. Mineral., 35, (2008), 163-174
Ottolini L. P., Le Févre B.: SIMS analysis of chlorine in metasomatised upper-mantle rocks, Microchim. Acta, 161, (2008), 329-336.
Matarrese S., Schingaro E., Scordari F., Stoppa F., Rosatelli G., Pedrazzi G., Ottolini L.: Crystal chemistry of phlogopite from Vulture-S.Michele Subsynthem volcanics (Mt.Vulture, Italy) and volcanological implications, Amer. Mineral., 93, (2008), 426-437.
Raffone N., Ottolini L., Tonarini S., Gianelli G., Fridleifsson G.O.: A SIMS study of lithium, boron and chlorine in basalts from Reykjanes (southwestern Iceland), Microchim. Acta, 161, (2008), 307-312.
Ottolini L., Laporte D., Raffone N., Devidal J.-L., Le Fèvre B.: New experimental determination of Li and B partition coefficients during upper mantle partial melting, Contrib.Mineral. Petrol., 157, (2009), 313-325.
Fregola R.A., Capitani G.C., Scandale E., Ottolini L.: Chemical control of 3T stacking order in a Li-poor biotite mica, Amer. Mineral., 94, (2009), 334-344.
Folco L., D’Orazio M., Tiepolo M., Tonarini S., Ottolini L., Perchiazzi N., Rochette P., Glass B.P.: Transantarctic Mountain microtektites: Geochemical affinity with Australasian microtektites, Geochim. Cosmochim. Acta, 73, (2009), 3694-3722.
Alletti M., Baker D.R., Scaillet B., Aiuppa A., Moretti R., Ottolini L.: Chlorine partitioning between a basaltic melt and H2O–CO2fluids at Mount Etna, Chem. Geol., 263, (2009), 37-50.
Ottolini L. P., Schingaro E., Scordari F., Mesto E., Lacalamita M.: The role of SIMS in the investigation of the complex crystal-chemistry of mica minerals, IOP (Institute of Physics) Conference Series: Materials Science and Engineering, EMAS 2009 – 11th European Workshop on Modern Developments and Applications in Microbeam Analysis, ISSN 1757-8981 (Print), ISSN 1757-899X (Online), (2010), doi: 10.1088/1757-899X/7/1/012023.
Raffone N., Ottolini L. P., Tonarini S., Gianelli G., D’Orazio M., Fridleifsson G. Ó.: An investigation of trace and isotope light elements in mineral phases from well RN-17 (Reykjanes Peninsula, SW Iceland), IOP (Institute of Physics) Conference Series: Materials Science and Engineering, EMAS 2009 – 11th European Workshop on Modern Developments and Applications in Microbeam Analysis, ISSN 1757-8981 (Print), ISSN 1757-899X (Online), (2010), doi: 10.1088/1757-899X/7/1/012026.
Galuskina I. O., Ottolini L., Kadiyski M., Armbruster T., Galuskin E. V., Dzieržanowski P., Winiarski A.: Pertsevite-(OH), a new mineral in the pertsevite series, Mg2(BO3)1–x(SiO4)x(F,OH)1–x (x < 0.5), from the Snezhnoye deposit in Sakha-Yakutia Republic, Russia, Amer. Mineral., 95, (2010), 953–958.
Groat L.A., Rossman G.R., Dyar M.D., Turner D., Piccoli P.M.B., Schultz A.J., Ottolini L.: Crystal chemistry of dark blue aquamarine from the true blue showing, Yukon Territory, Canada, Canad. Mineral., 48, (2010), 597-613.
Scordari F., Dyar M.D., Schingaro E., Lacalamita M., Ottolini L.: XRD, micro-XANES, EMPA, and SIMS investigation on phlogopite single crystals from Mt. Vulture (Italy), Amer. Mineral., 95, (2010), 1657-1670.
Clark C.M.C., Hawthorne F.C., Ottolini L.: Fluor-dravite, NaMg3Al6Si6O18(BO3)3(OH)3F, a new mineral species of the tourmaline group from the Crabtree Emerald Mine, Mitchell County, North Carolina: description and crystal structure, Canad. Mineral., 49, (2011), 57-62.
Hatert F., Ottolini L., Schmid-Beurmann P.: Experimental investigation of the alluaudite + triphylite assemblage, and development of the Na-in-triphylite geothermometer: applications to natural pegmatite phosphates, Contrib. Mineral. Petrol., 161, (2011), 531-546.
Manuella F.C., Carbone S., Ottolini L., Gibilisco S. : Micro-Raman spectroscopy and SIMS characterization of oxykinoshitalite in an olivine nephelinite from the Hyblean Plateau (Sicily, Italy), Eur. J. Mineral., 24, (2012), 527-533.
Ottolini L., Schingaro E., Scordari F.: Contribution of Secondary Ion Mass Spectrometry (SIMS) to the Study of Crystal Chemistry of mica minerals, Mass Spectrometry Handbook, Chapter 43/Ceramics, (2012), 1017-1059, Ed. Mike S. Lee, JOHN WILEY & SONS, INC., PUBLICATION.
Aurisicchio A., Conte A.M., De Vito C., Ottolini L.: Beryl from miarolitic pockets of granitic pegmatites, Elba, Italy: characterization of crystal chemistry by means of EMP and SIMS analyses, Canad. Mineral., 50, (2012), 1467-1488.
Hatert F., Ottolini L., Wouters J., Fontan F.: A structural study of the lithiophilite- sicklerite series, Canad. Mineral., “ Granitic pegmatites and their minerals: A tribute to Petr Cerny ”, 50(4), (2012), 843-854.
Schmid-Beurmann P., Ottolini L., Hatert F., Geisler T., Huyskens M., Kahlenberg V.: Topotactic formation of ferrisicklerite from natural triphylite under hydrothermal conditions, Mineral. Petrol., 107, (2013), 501-515.
Rao C., Wang R., Hatert F., Gu X., Ottolini L., Hu H., Dong C., DalBo F., Baijot M.: Strontiohurlbutite, SrBe2(PO4)2, a new mineral from Nanping No. 31 pegmatite, Fujian Province, Southeastern China, Amer. Mineral., 99, (2014), 494-499.
Ottolini L.P., Scordari F., Mesto E.: A new application of SIMS to the analysis of nitrogen in mica minerals: tobelite, IOP Conference Series: Materials Science and Engineering Vol. 55, conference 1, (2014), EMAS 2013 Workshop: 13th European Workshop on Modern Developments and Applications in Microbeam Analysis, 012014 doi:10.1088/1757-899X/55/1/012014.
Laporte D., Lambart S., Schiano P., Ottolini L.: Experimental derivation of nepheline syenite and phonolite liquids by partial melting of upper mantle peridotites, Earth Planet. Sci. Lett., 404, (2014), 319–331.
Gentili S., Bonadiman C., Biagioni C., Comodi P., Coltorti M., Zucchini A., Ottolini L.: Oxo-amphiboles in mantle xenoliths: evidence for H2O-rich melt interacting with the lithospheric mantle of Harrow Peaks (Northern Victoria Land, Antarctica), Miner. Petrol., 109, (2015), 741-759.
Schingaro E., Lacalamita M.., Mesto E., Ventruti G., Pedrazzi G.., Ottolini L., Scordari F..: Crystal chemistry and light elements analysis of Ti-rich garnets, Amer. Mineral., 101, (2016), 371-384.
Balassone G., Bellatreccia F., Ottolini L., Mormone A., Petti C., Ghiara M.R., Altomare A., Saviano M., Rizzi R., D'Orazio L.: Sodalite-group minerals from Somma-Vesuvius volcano (Naples, Italy): A combined EPMA, SIMS and FTIR crystal chemical study, Can.Mineral. 2015 canmin.1500083; published ahead of print December 23, 2015, doi:10.3749/canmin.1500083.