Melt inclusions and Volcanic Shards
The high spatial resolution and sensitivity of SIMS in the analysis of trace, light and volatile elements in geologic samples are particularly requested in the investigation of melt inclusions. These latter are small quantities of magma included in minerals during coarsening, growth or recrystallisation of the crystal structure. They can be found in various types of minerals and their morphology varies from a host mineral to another, often subordinate to their host mineral crystallography or else repeating its form.
Melt inclusions sample small volumes of the parental liquids trapped during growth of their host phases and thus their compositions record intermediate steps in the evolution of magmas. In particular, melt inclusions in the early-crystallised minerals in basalts have been proposed to be pristine samples of high-pressure mantle-derived melts that were trapped prior to mixing at shallower levels. Therefore, their ability to preserve and record the geochemical evolution provides with a highly adapted tool to detail the different processes that governed the magma genesis from its formation in the mantle to its emission at the earth surface.
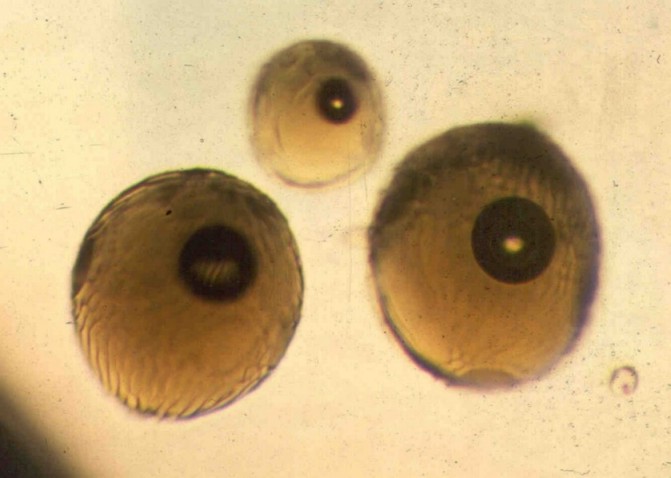
Fig. 1- Melt inclusions in a crystal of olivine. Their dimensions typically range from few microns to few tens of microns (courtesy of P. Schiano & R. Clocchiatti). |
The geodynamic setting of the Southern Tyrrenian-Sicilian region has drawn the attention of many investigators since its evolution is accompanied by two radically different types of volcanism, one essentially calc-alkaline magmatism found in the Aeolian Islands (including Vulcano Island), and another of basaltic affinity typical of the nearby Eastern Sicily volcanoes (Mount Etna and Mts Iblei). Mount Etna and Iblean plateau are sided on the suture between the converging European and African plates, adjacent to the Aeolian arc. The almost contemporaneous existence of alkaline-type and calc-alkaline type volcanism and also the absence of seismic evidence for an active Benioff zone directly beneath Sicily place a number of constraints regarding the geodynamic picture of the Southern Tyrrhenian Sea. A number of (often controversial) tectonic environment models have been proposed for source of the volcanism of alkaline affinity found at Mount Etna. Arguments in support of these different models, however, suffer the paucity of information relative to the primary composition of magmas from Mount Etna.
The ion microprobe at the SIMS lab in Pavia has been used to characterise the source chemistry of Vulcano Island, Mount Etna and Mts Iblei volcanisms by examining the trace-element composition of primitive magmas, preserved as primary inclusions in magnesian olivine phenocrysts.
For Vulcano, the melt inclusions in phenocrysts of rocks of the shoshonitic eruptive complex are characterised by high Ca/Al ratio and present very similar Rb/Sr, B/Be and patterns of trace elements, with Nb and Ti anomalies typical of a subduction zone.
Together with an essentially calc-alkaline magmatism found in the Aeolian Islands, a magmatism of basaltic affinity coexists, expressed in the nearby Eastern Sicily volcanoes (Mts Iblei and Mt Etna).
For Mt Etna, the ion microprobe data indicate that primitive magmas trapped in olivines from Aci Castello (500,000 yr) and those of Ancient Alkaline Centers (> 100,000 yr) have compositions similar to those of the Iblean Mountains. Over times changes occur in the chemical composition of Mount Etna primitive magmas in the range 100,000 and 7,000 yr. When plotted in variation diagrams, the 1999 melt inclusions (SE Crater) display strong similarities with Mount Maletto (7,000 yr) melt inclusions, and their trace element patterns and incompatible element ratios are intermediate between typical melt inclusions from Vulcano island (La Sommata) and Mts Iblei. Various extents of mixing between melts of the two sources (Vulcano-type source and Mts Iblei-type source) can account for both the global chemical evolution of the Etnean primary magmas through time and the characteristic features (strong enrichments in alkalis, with accompanying increases of 87Sr/86Sr ratios) of the present-day and recent (post-7000 years) lavas.
Because the composition of a primary magma is a direct reflection of its source, the results imply that the source of Mount Etna has changed over time: the compositional change in primary magmas from Mt. Etna reflects a progressive transition from a predominantly mantle-plume source to one with a greater contribution from island-arc (subduction-related) basalts (Schiano et al., 2001).
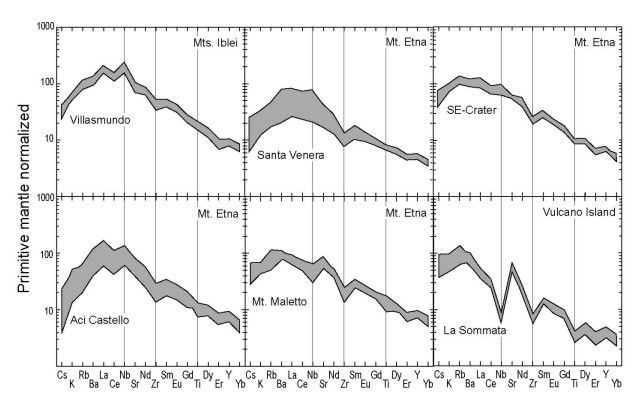
Fig. 2- Ranges of trace-element compositions (normalised to primitive mantle) for olivine-hosted melt inclusions in basalts from Mount Etna: Aci Castello (520 kyr), Santa Venera 120 kyr), Mount Maletto (7 kyr) and the 1999 southeast (SE) crater series; Vulcano island (La Sommata) and the Iblean Mountains (Villasmundo) (Schiano et al., 2001). |
These results have important implications in the view of future explosive events on Mount Etna, in understanding the geodynamics of Southern Tyrrenian sea.
The relationship between potassic, calc-alkaline and Na-alkaline magmatism in South Italy Volcanoes has been investigated by means of a melt inclusion approach (Schiano et al., 2004) using the ion microprobe.
Trace-element relationships of olivine-hosted melt inclusions in basalts from South Italy volcanoes (Vesuvius, Phlegraean Fields, Stromboli,Vulcano and Ustica) confirm the existence of large mantle heterogeneity in this area. Mantle sources for the Aeolian Islands and the Campania Province melt inclusions originate from subduction-related metasomatic processes, whereas the source for Ustica Island melt inclusions stems from the upwelling of mantle materials. Even though they are found in two subduction settings, primitive melt inclusions from Stromboli-Vulcano and Vesuvius-Phlegraean Fields volcanoes show differences that can simply be accounted for by considering the evolution of a subducted oceanic crust and the depth at which the various metasomatic and melting processes take place. At shallow to intermediate depths, aqueous fluids are derived from dehydratation of K-free phases because of the extended K-mica (phengite) stability. These fluids could then act as the metasomatic agents for the generation of calc-alkaline magmas such as those recorded in Stromboli and Vulcano melt inclusions. At larger depths, K-rich fluids or melts are released from the subducting oceanic crust during dehydratation or melting processes. They rise to produce in the overlying mantle phlogopite or K-rich amphibole (by metasomatism or hybridisation reactions), which may subsequently contribute to potassic volcanism similar to that of Vesuvius and the Phlegraean Fields.
In contrast, olivine-hosted melt inclusions from Ustica magmatism display compositions remarkably similar to olivine-hosted melt inclusions from Eastern Sicily volcanoes. They are consistent with derivation from an enriched intraplate mantle source similar to that of the Eastern Sicily volcanoes; i.e. they are characterised by a signature that is likely to reflect an ancient basaltic source component. Moreover, their trace-element features suggest that the interaction process between the intraplate-arc sources recorded at Mount Etna is also operating beneath Ustica Island, about 60 km North of the Sicilian coast.
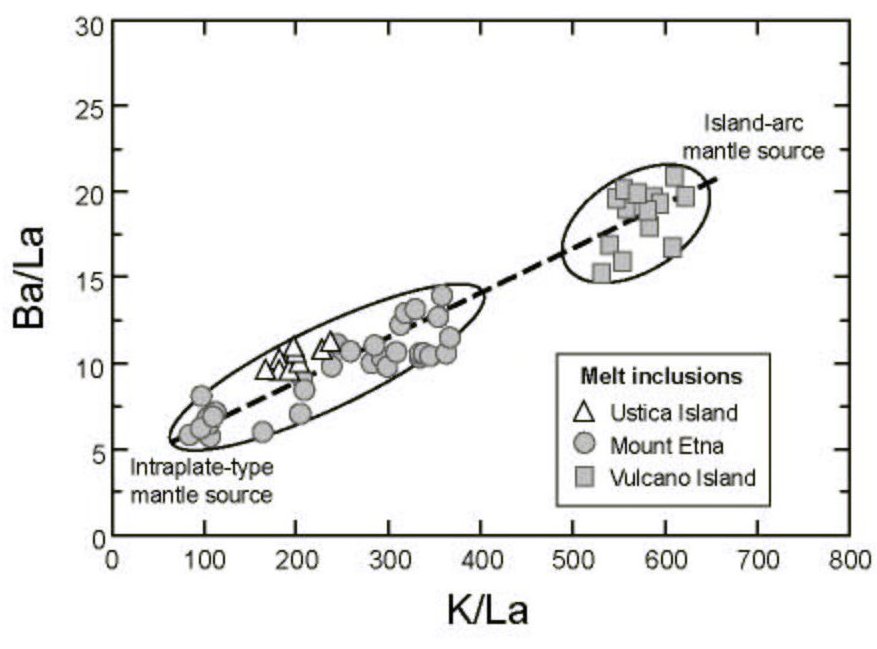
Fig. 3- Ba/La vs. K/La diagram comparing Ustica Island melt inclusions with olivine-hosted melt inclusions from Mount Etna and Vulcano Island. Ustica Island melt inclusions plot along the mixing trend defined by Mount Etna primary magmas, suggesting that the interaction process between the interplate-arc sources recorded at Mount Etna is also operating beneath Ustica Island. Shown for comparison is the estimated composition of the sources of HIMU, EM1 and EM2-type OIB. |
Major- and trace-element abundances have been obtained for olivine-hosted melt inclusions from a picritic basalt (ARP73–10–03) dredged in the FAMOUS locality along the Northern Mid-Atlantic Ridge. Isolated in homogeneous, highly primitive olivine phenocrysts (Fo87.2–91.3), melt inclusions provide important information about the processes of melt generation beneath mid-ocean ridges. They contain near-primary magmas that display a large range of major- and trace-element compositions and form a primitive continuation of the FAMOUS whole rock suites. Both slightly depleted and enriched melt inclusions can be distinguished on the basis of their trace-element compositions, with (La/Sm)N ratios ranging from 0.58 to 1.52.
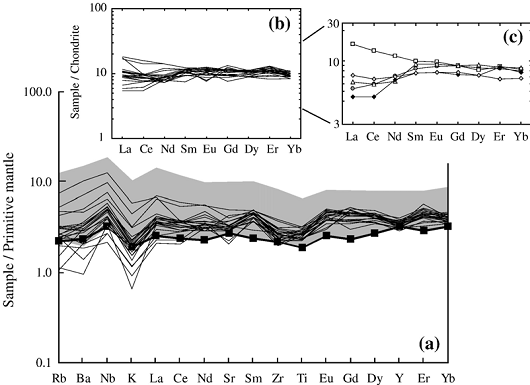
Fig. 4- Primitive mantle-normalized diagram (Sun and McDonough, 1989) (a) showing trace-element data for olivine-hosted melt inclusions in MORB sample ARP73–10–03, obtained at CNR-IGG (Pavia). The elements are classified according to the incompatibility sequence previously determined for MORB genesis (Hofmann, 1988). Also shown for comparison are the compositions of FAMOUS whole rocks and basalt glasses (shaded area; Langmuir et al., 1977; White and Bryan, 1977; Le Roex et al., 1981) and the composition of the sample ARP73–10–03 used for this study (solid squares). The insert (b) shows the chondrite C1- (Sun and McDonough, 1989) normalized abundance patterns for rare-earth elements in the melt inclusions. Crossing of REE patterns in the melt inclusion data is illustrated by five representative compositions (c) (Laubier et al., 2007). |
Melt inclusions display chemical trends on both major- and trace-element variation diagrams, indicating that they constitute a suite of genetically-related, highly primitive magmas. The evolution of the melt inclusions is inconsistent with a model of mantle-melt chemical exchange during reactive transport, suggesting that the trapped melts are able to preserve pristine information about the melting process and/or source composition. Comparison of these trends with predicted curves for models of peridotite melting indicates that compositional variations are best reproduced by polybaric partial melting of a relatively homogeneous mantle sourceand subsequent mixing in various proportions of the melt batches produced at different degrees of melting and/or in different parts of the melting system. These observations require that transport of melts from the melting region to the site of olivine crystallization occurs without significant chemical exchange with the surrounding mantle (Laubier et al., 2007).
The SIMS technique was used to investigate -at our knowledge, for the first time- the melt inclusions trapped within early-formed olivine phenocrysts from Marsili seamount, a large volcano in the active Marsili back-arc basin of the Southern Tyrrhenian. Trace element data not only shed light on the petrogenesis of Marsili magmatism, but also provided a better understanding of the origin of the wide spectrum of magma-types occurring in that area, as well as their relationship with the geodynamic evolution of the region. The large range in incompatible trace element abundances of the Marsili melt inclusions is consistent with a melting scenario involving different mantle sources, recording a complex mixture of silicate liquids derived from different mantle sources in the mantle wedge and variously contaminated by components (fluid or melt) released from the subducting slab (Trua et al., 2010).
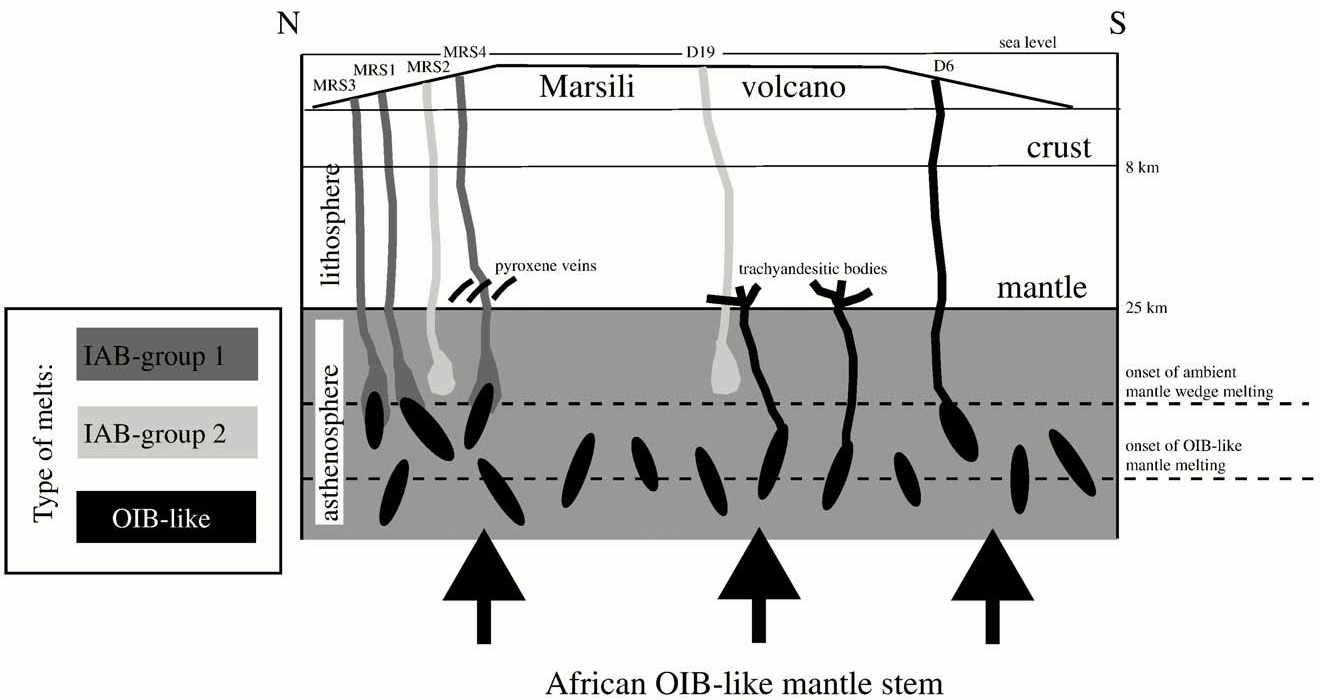
Fig. 5- Cartoon illustrating possible melting mechanism of the heterogeneous Southern Tyrrhenian asthenospheric mantle beneath Marsili volcano. Small-scale heterogeneity within the asthenospheric mantle is related to the enriched OIB-like mantle that flowed into the mantle wedge melting region. We suggest that the locus of melting and the subsequent fractal melt extraction strongly controls the composition of the erupted Marsili mafic magmas: OIB-like melts were generated at greater depth, from portions of the OIB-like mantle; group 2-IAB type Marsili lavas derived from the melting, at shallower depths, of the ambient MORB-type subduction-metasomatized mantle wedge; group 1-IAB type Marsili lavas also formed once the heterogeneous mantle crossed the solidus of the ambient mantle wedge, thus permitting a variable mixture of melts derived from the OIB-like and the ambient mantle wedge components (see Trua et al., 2010 for further details). |
Chromite ores formation is still a debated topic. In the study carried out by Merlini et al. (2011), detailed analyses of major, minor elements by EMPA, and Rare Earth Elements (REE) by SIMS, were performed on silicate inclusions detected in chromite grains of chromitite pods, enveloping dunites and on clinopyroxene of lherzolitic host rock of well known Trodoos chromite ores. The detailed study of included clinopyroxene at very small scale, using accurate EMPA and SIMS methods, demonstrates the power of clinopyroxene mineral chemistry. It can be a helpful tool not only for the understanding of petrogenetic processes acting in ophiolite mantle sequences and chromite ore formation, but also to help exploration of chromite ores within ophiolite mantle as its geochemical signature provides useful information to distinguish between chromite ore fertile (Dunite1 type) and barren (Dunite2 type) dunites.
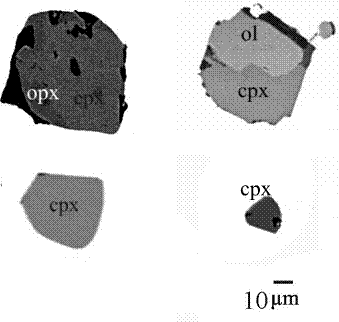
Fig. 6- Back Scattered Image of chromite grains hosting different types of silicate inclusions; cpx=clinopyroxene, opx=orthopyroxene, ol=olivine (Merlini et al., 2011). |
Tephra layers preserved in marine sediments can contribute to the reconstruction of volcanic histories and potentially act as stratigraphic isochrons to link together environmental records. Recent developments in the detection of volcanic ash (tephra) at levels where none is macroscopically visible (so called ‘crypto-tephra’) have greatly enhanced the potential of tephrostratigraphy for synchronising environmental and archaeological records by expanding the areas over which tephras are found.
In the paper by Satow et al. (2015), crypto-tephra extraction techniques allow the recovery of 8 non-visible tephra layers to add to the 9 visible layers in a marine sediment core (LC21) from the SE Aegean Sea to form the longest, single core record of volcanic activity in the Aegean Sea. Using a novel, shard-specific methodology, sources of the tephra shards are identified on the basis of their major and trace element single-shard geochemistry, by comparison with geochemical data from proximal Mediterranean volcanic stratigraphies. The results indicate that the tephra layers are derived from 14 or 15 separate eruptions in the last ca 161 ka BP: 9 from Santorini; 2 or 3 from Kos, Yali, or Nisyros; 2 from the Campanian province; and one from Pantelleria.
The attributions of these tephra layers indicate that 1) inter-Plinian eruptions from Santorini may have produced regionally significant tephra deposits, 2) marine tephrostratigraphies can provide unique and invaluable data to eruptive histories for island volcanoes, and 3) tephra from both Pantelleria and Campania may be used to correlate marine records from the Aegean Sea to those from theTyrrhenian, Adriatic and Ionian Seas.
Tephra LC21 (4.285) also is a sample from a peak in cryptotephra. The shards are often very small (<40 µm diameter) and have very large elongate vesicles within them, making them difficult to analyse using the available geochemical techniques. They do not show any evidence of geochemical alteration. The results of 11 EPMA measurements define the shards as rhyolitic. The shape and size of the glass surfaces on the shards made them impossible to analyse on the 25 µm diameter LA-ICP-MS system but the 5 µm beam of the SIMS apparatus of the Cameca IMS 4f ion microprobe at CNR-IGG, Pavia allowed five quantitative assessments of trace element concentrations. Nb ranges from 19.6 to 23.0 ppm while Zr is more depleted with values of 161-206 ppm (see Fig. 7). The rhyolitic chemistry of the shards in this sample is consistent with both of these sources. The LC21 age model suggests an age for LC21 (4.285) of between 32.894 ± 0.502 and 32.992 ± 0.503 ka BP.
This study is therefore the first to discover crypto-tephra originating from one of these sources, extending the known footprint of these two volcanoes to approximately 100 km southwest of the islands (see Satow et al., 2015 for details).
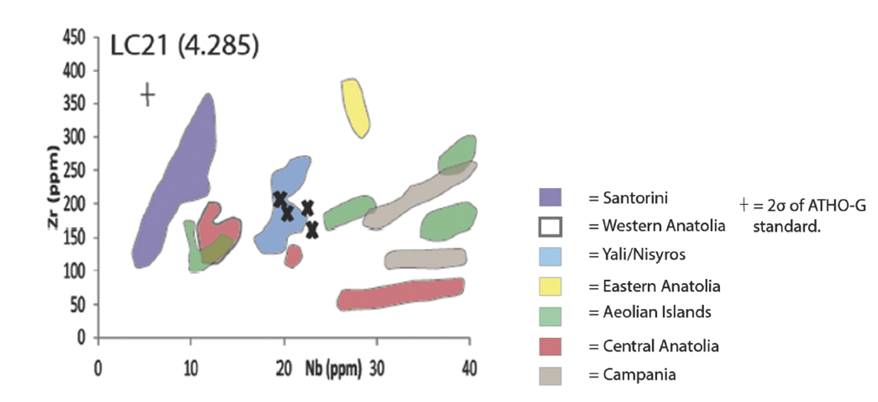
Fig. 7- The rhyolitic chemistry of the shards in this sample, and the concentrations of Zr and Nb are consistent with a source from the Yali or Nisyros centres. |
A new application of our Cameca IMS 4f ion microprobe concerned the investigation of trace elements in volcanic shards from Y-3 tephra, a crucial and widespread stratigraphic marker within the central Mediterranean region. It offers enormous potential to assess potential leads and lags between Mediterranean archives in response to abrupt climatic shifts.
Until now the type locality Y-3 tephra has remained poorly characterised. Consequently numerous other distal tephras occurring in broadly similar stratigraphic positions, displaying a similar K-trachytic chemistry, have been correlated to the Y-3 without precise geochemical validation. In the paper by Albert et al. (2015) we present the first grain specific multi-elemental characterisation (EPMA and LA-ICP-MS) of the Y-3 tephra recorded in its type locality of the Ionian Sea. In addition, SIMS (ion probe) technique at CNR-IGG, Pavia was used, for the first time in sample like this, to get accurate trace-element patterns in very tiny (= 10 microns) shards of the sample set. The High-K phono-trachyte geochemistry of the type locality Y-3 supports a provenance from within Campi Flegrei caldera, consistent with previous interpretations. The precise provenance of the Ionian Sea Y-3 tephra is assessed in the context of the intra-caldera and extra-caldera Tufi Biancastri deposits. Using the diagnostic major and trace element chemistry of the type locality Y-3 tephra Albert et al. (2015) revised and established precise distal-distal correlations. Robust identification of Y-3 in Tenaghi Philippon (Greece) both extends its eastern dispersal and offers an opportunity to determine a precise age for this distal tephra. Through the identification of the diagnostic Y-3 glass chemistry this tephra can provide a crucial tephrostratigraphic and chronostratigraphic marker facilitating the precise synchronisation of marine and lacustrine palaeoenvironmental archives over significant distances at a crucial environmental transition.
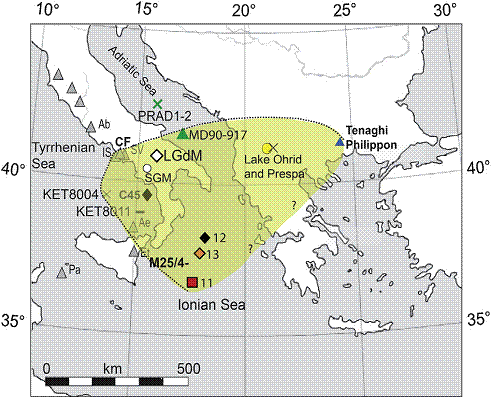
Fig. 8- The revised known dispersal and distribution of Y-3 tephra (Albert et al., 2015). |
Sites in North Africa hold key information for dating the presence of Homo sapiens and the distribution of
Middle Stone Age (MSA), Middle Palaeolithic (MP) and Later Stone Age (LSA) cultural activity in the Late Pleistocene. In the paper by Barton et al. (2015), we present new and review recently published tephrochronological evidence for five cave sites in North Africa with long MSA/MP and LSA cultural sequences. Four tephra horizons have been identified at the Haua Fteah (Cyrenaica, Libya). They include cryptotephra evidence for the Campanian Ignimbrite (CI) eruption dating to ~39 ka that allows correlation with other Palaeolithic sequences in the eastern Mediterranean and as far north as Russia. Cryptotephra have also been recorded from the Moroccan sites of Taforalt, Rhafas and Dar es-Soltane 1. Our ion microprobe Cameca IMS 4f, installed at CNR-IGG (Pavia), was used successfully to quantify the trace element single-grain glass shard compositions for cryptotephra horizons TAF_S1_R2 and TAF_S8_Y1, some of which of very small size (< 10 micron Ø). Although the proximal volcanic sources for cryptotephra layers in Taforalt are at present imprecisely known, they provide strong circumstantial evidence for long distance transport of tephra shards from volcanoes on the Azores. In each of these instances the tephra signal is sufficiently strong to show the potential for extending this approach to other terrestrial sites from across North Africa to the Levant, as well as adjacent offshore marine deposits (see Barton et al., 2015 for details).
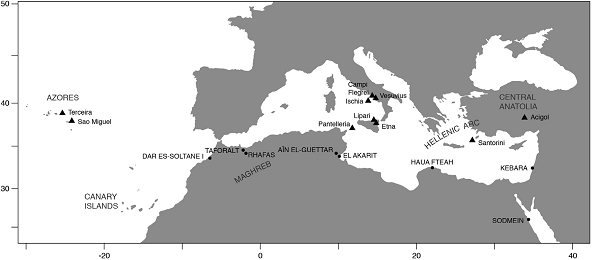
Fig. 9- Distribution of study sites in North Africa and the Levant, and volcanic centres discussed in the paper by Barton et al. (2015). |
Mineral-hosted silicate melt inclusions (MIs) have the potential to preserve information on the instantaneous composition and evolutionary conditions of magmatic systems, which is completely or partially obliterated in bulk-rock or glass chemistries by secondary processes such as contamination, melt mixing, crystal fractionation, and degassing [e.g., Roedder, 1984; Sobolev, 1996]. MIs trapped by growing crystals at different stages of magma evolution may provide crucial chemical information on mantle components involved in the present-day Etnean activity, as well as document localized, grain-scale processes within the plumbing system. In the paper by Schiavi et al., 2015, we present the results of a comprehensive study of major, trace elements, and lead isotopes of phenocryst-hosted MIs from the 2001–2006 Etna eruptions to better understand the nature of the mantle sources and highlight the processes occurring at crustal levels. The new data are discussed in terms of possible source heterogeneity, changes in magma sources through time and/or processes linked to magma dynamics within the deep and shallow plumbing system.
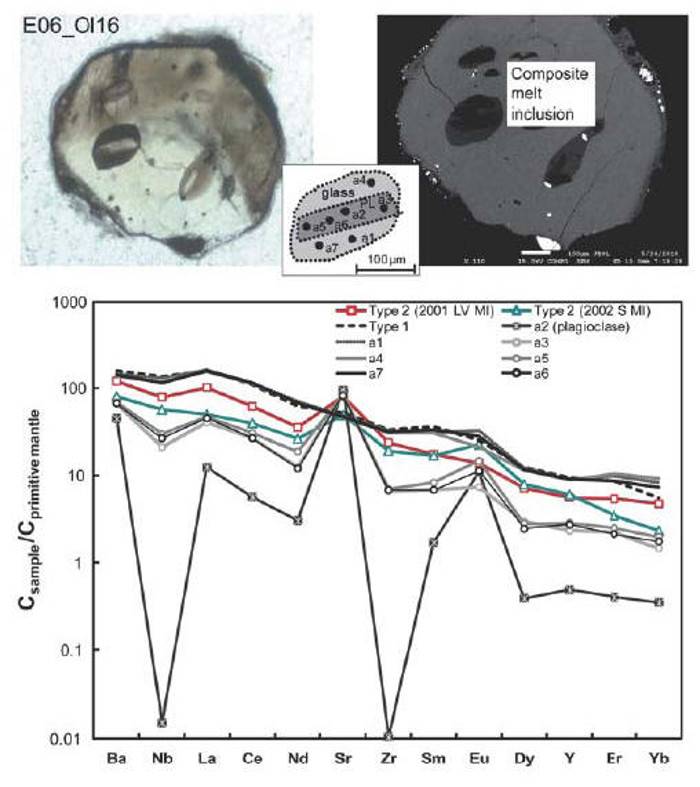
Fig. 10- (as Fig. S6 from Supporting Information of the paper Schiavi et al. (2015). Olivine-hosted composite MI from 2006 sample: under the optical microscope (top left), SEM image of the same (top right; note the large plagioclase crystal, and schematic cartoon of the inclusion with spot positions (top centre). Bottom: Primitive-mantle-normalized trace element patterns of the seven spot analyses, obtained with the ion microprobe Cameca IMS 4f at CNR-IGG (Pavia). Patterns of a1, a4 and a7 glass spots closely resemble that of Type-1 MIs. Patterns of a3, a5 and a6 spots result from 20-30% addition of Type-1-like melt to plagioclase, whereas the compositions of Type-2 MIs require lower plagioclase addition (<50%) to Type-1-like melt. 2002 S MI is that identified as Type-2 end-member (L6-1) in Fig. 3 of Schiavi et al. (2015) |
References
Gioncada A., Clocchiatti R., Sbrana A., Bottazzi P., Massare D., Ottolini L.: A study of melt inclusions at Vulcano (Aeolian Islands, Italy): insights on the primitive magmas and on the volcanic feeding system, Bull. Volcanol., 60, (1998), 286-306.
Clocchiatti R., Schiano P., Ottolini L., Bottazzi P.: Earlier alkaline and transitional magmatic pulsation of Mt Etna Volcano, Earth Planet. Sci. Lett., 163, (1998), 399-407.
Schiano P., Clocchiatti R., Ottolini L., Busa’ T.: Transition of Mount Etna lavas from a mantle-plume to an island-arc magmatic source, Nature, 412, (2001), 30 August Issue, 900-904.
Kassaa S., Laridhi-Ouazaa N., Cbaftar R., Massare D., Ottolini L., Clocchiatti R.: Evolution de la composition des reliquats magmatiques (RM) des cristaux de quartz au cours de refroidissement du dôme de rhyodacites de l’oued Belif (Tunisie): un exemple des limites et possibilités d’utilisation des IV dans les interprétations pétrogénétiques, Réunion des Sciences de la Terre, Nantes, 9-12 Avril, (2002), 256, p. 150.
Schiano P., Clocchiatti R., Ottolini L., Sbrana A.: The relationship between potassic, calc-alkaline and Na-alkaline magmatism in South Italy volcanoes: A melt inclusion approach, Earth Planet. Sci. Lett., 220, (2004), 121-137.
Laubier M., Schiano P., Doucelance R., Ottolini L., Laporte D.: Olivine-hosted melt inclusions and melting processes beneath the FAMOUS zone (Mid-Atlantic Ridge), Chem. Geol., 240, (2007) 129–150.
Trua T., Clocchiatti R., Schiano P., Ottolini L., Marani M.: The heterogeneous nature of the Southern Tyrrhenian mantle: Evidence from olivine-hosted melt inclusions from back-arc magmas of the Marsili seamount, Lithos, 118, (2010) 1-16.
Merlini A., Grieco G., Ottolini L., Diella V.: Probe and SIMS investigation of clinopyroxene inclusions in chromites from the Troodos chromitites (Cyprus): implications for dunite-chromitite genesis, Ore Gelogy Reviews, 41, (2011) 22-34.
Satow C., Tomlinson E.L., Grant K.M., Albert P.G., Smith V.C., Manning C.J., Ottolini L., Wulf S., Rohling E.J., Lowe J.J., Blockley S.P.E., Menzies M.A.: A new contribution to the Late Quaternary tephrostratigraphy of the Mediterranean: Aegean Sea core LC21, Quaternary Science Reviews, 117, (2015) 96-112.
Albert P.G., Hardiman M., Keller J., Tomlinson E. L., Smith V.C., Bourne A.J., Wulf S., Zanchetta G., Sulpizio R., Müller U.C., Pross J., Ottolini L., Matthews I.P., Blockley S.P.E., Menzies M.A.: Revisiting the Y-3 tephrostratigraphic marker: a new diagnostic glass geochemistry, age estimate, and details on its climatostratigraphical Context, Quaternary Science Reviews, 118, (2015) 105-121.
Barton R.N.E., Lane C.S., Albert P.G., White D., Collcutt S.N., Bouzouggar A., Ditchfield P., Farr L., Oh A., Ottolini L., Smith V.C., Van Peer P., Kindermann K.: The role of cryptotephra in refining the chronology of Late Pleistocene human evolution and cultural change in North Africa, Quaternary Science Reviews, 118, (2015) 151-169.
Schiavi F., Rosciglione A., Kitagawa H., Kobayashi K., Nakamura E., Nuccio P.M., Ottolini L., Paonita A., Vannucci R.: Geochemical heterogeneities in magma beneath Mount Etna recorded by 2001–2006 melt inclusions, Geochemistry, Geophysics, Geosystems (G3), 16, 21 May 2015, doi:10.1002/2015GC005786.