Other Trace Elements
A SIMS procedure that relies on "energy filtering" and on the empirical approach to quantification has been also developed for the analysis of other (trace) elements of relevant geochemical significance, such as Y, Ti, V, Cr, Zr, Sr, Nb in (ortho and clino)- pyroxenes, amphiboles and garnets. 45Sc+, 47Ti+, 51V+, 52Cr+, 88Sr+, 89Y+, 90Zr+ and 93Nb+ are their isotopes detected. 30Si+ is chosen as the inner reference for the matrix. Sc and Cr are further corrected for the presence of residual interferences due to 29Si16O+ and 24Mg28Si+, respectively.
Moreover, elements, such as K (as 39K+), Cs (as 133Cs+), Ba (as 137Ba+ or 138Ba+), Rb (as 85Rb+) are currently analysed in amphibole, and Sr and Ba (V, Zr) are analysed in plagioclase. Residual interferences at 85Rb+ are checked through low-Rb standards.
Na (as 23Na+), K, Cs, Rb, Ba, Hf (as 178Hf+ and 180Hf+), U (as 238U+), Th (as 232Th+) and Ta (as 181Ta+) can be measured in several silicates.
The quantification of REE, Sr, Ba, U, Th, …. can be done also in apatites and other phosphates, Durango, Mud Tank and Snarum being the standards employed (courtesy of S.J.B. Reed and N.D. MacRae).
The samples used for calibration of ion signals in cpx, opx e plg are the international cpx standards KH1 (Kilbourne Hole) and Kakanui augite (KAUG), whereas Kakanui (KAKG) and MNAG pyrope represent our reference samples for gnt (courtesy of N. Shimizu).
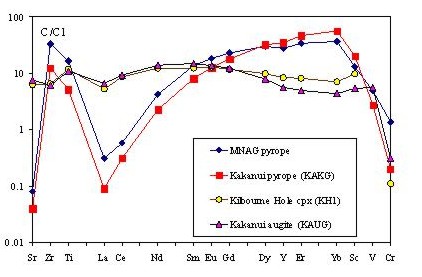
Fig. 1- Fig. 1- C1-chondrite normalised trace-element patterns of our standards for SIMS analysis in gnt, px and plg. |
Quantification of trace elements in amph relies mainly on Kakanui amphibole (courtesy of N. Shimizu), kaersutite Soda Spring (KSS) (courtesy of F. Caporuscio), and several other well-developed standards. We use also the international synthetic glasses of NIST-6XX series (i.e., NIST-610, 612), BCR-2G (from USGS) and well-characterised samples such as the basaltic glass BB (courtesy of K. Schmidt). The amphibole standards are used also for some trace elements in micas. Precision and accuracy are in the range 5-15% for all the elements at ppm (wt) level, and = 25% for Rb.
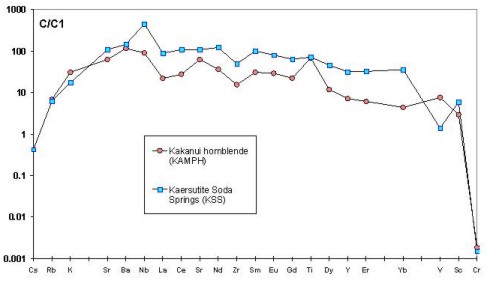
Fig. 2- C1-chondrite normalised trace-element patterns of our standards used for SIMS analysis in amphibole. |
In the paper by Vannucci et al. (1995), we show that LREE-, Sr-depleted kaersutite and Ti-pargasite are widely diffused in subcontinental mantle sectors, as documented by orogenic peridotites and lherzolite xenoliths. In the absence of experimental evidence that LREE-, Sr-depleted H2O-rich fluids could migrate from deep mantle, we propose either a diffusive redistribution or a chromatographic process to account for the crystallization of depleted amphibole from LREE-, Sr-enriched fluids. At least for some peridotite massifs (i.e., Zabargad, External Ligurides, Eastern Pyrenees), the textural evidence indicates that the disseminated accessory amphibole was formed before the injection of alkaline pyroxenites and homblendite veins that date the initial stages of rifting. The crystallization of LREE-, Sr-depleted kaersutite and Ti-pargasite is, therefore, related to the early evolution of these peridotite bodies and seems to characterize the steady-state equilibration under spinel-facies conditions. In the geotectonic scenario depicted for the Ligurian and Red Sea subcontinental mantle, this stage is broadly related to the timing of lithospheric accretion. It seems, in fact, plausible that, after the early upwelling of asthenospheric mantle, fluids were released from deeper mantle sectors and extensively penetrated the lithosphere, causing amphibole crystallization and probably partially restoring refractory material to more fertile compositions.
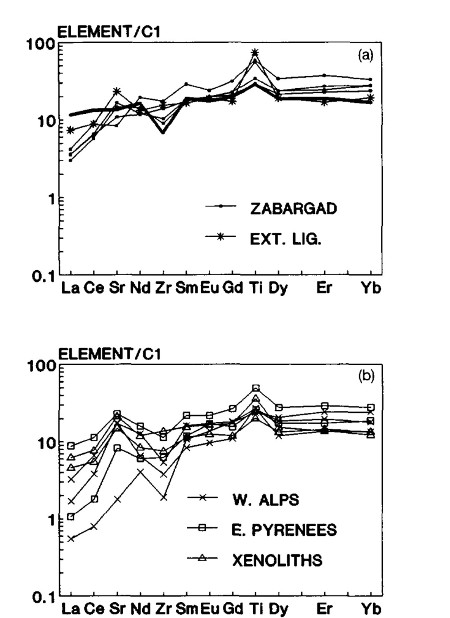
Fig. 3- Spidergrams for the investigated kaersutites and Ti-pargasites. (a) Spinel-lherzolites from Zabargad (Red Sea) and External Ligurides (Northern Apennines); sample 22099 from Zabargad, which does not show evidence of partial recrystallization to plagioclase-bearing assemblage is reported in bold. (b) Spinel-lherzolites from Balmuccia and Premosello (Western Alps), Lherz and Freychinede (Eastern Pyrenees), and peridotite xenoliths from Hungary and Austria (see Vannucci et al., 1995 for details).
|
|
The SIMS technique was used in the characterization of trace elements (Li, Be, B, F, Sc, Ti, V, Cr, Rb, Sr, Zr, Nb, Cs and Ba) in phengites developed under ultrahigh-pressure (UHP) metamorphism from the UHP Brossasco-Isasca Unit (Dora Maira Massif, Italy) in a multiple-analytical study including Ar laser probe and TIMS to examine the behaviour of 40Ar-39Ar and Rb-Sr systems (Di Vincenzo et al., 2006). The ion-microprobe analysis of phengite in the marble revealed a pronounced zoning of trace elements, including Rb and Sr, which is in accord with major element zoning (see Fig. 3).
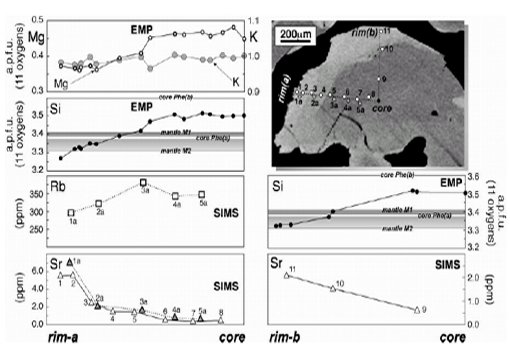
Fig. 4- Core to rim trace and major element variations in phengite grain. |
The SIMS and EMP results support a concomitant decrease in celadonite content and Rb/Sr ratio from the core to the rim of the phengite grain (Fig. 4), and indicate a decrease in the Rb/Sr ratio during exhumation. The inversion of aragonite to calcite during exhumation may have released significant amounts of Sr, such that the final stage of phengite chemical re-equilibration occurred in a Sr-rich systems as observed in other natural samples. The apparent negative correlation in Fig. 5is an artefact caused by imperfect mineral separation and, probably, by contamination with K-feldspar or epidote. Among the six phengite fractions analysed for the Rb-Sr systematics, only wet-ground fractions (1) and (2d-2), yielding isochron ages of 36.45 ± 0.36 and 41.1 ± 0.40 Ma and preserving the age vs. Rb/Sr ratio relationship (Fig. 5) defined by EMP-SIMS data, appear internally consistent (see Di Vincenzo et al., 2006, for details).
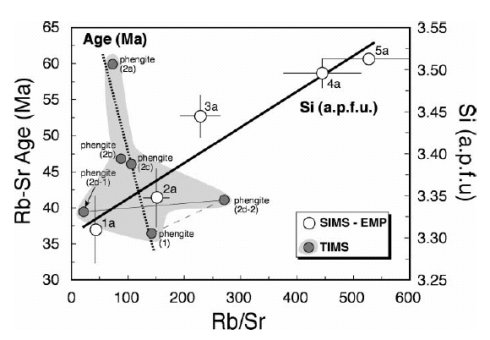
Fig. 5- Rb–Sr age and Si content (a.p.f.u.) vs Rb/Sr ratio for phengites of sample CM1. Numbers for SIMS–EMP data points refer to those reported for SIMS analysis in Fig. 4 and those for Rb–Sr data determined by TIMS (thermal ionization mass spectrometry) on the phengite fractions of Table 3 (see Di Vincenzo et al., 2006). The bold dotted line shows the trend defined by the finer-grained phengite fraction [phengite (1)] and the three unground phengite fractions [(2a), (2b) and (2c)] derived from the >0.50 mm grain size. The bold continuous line shows the trend defined by SIMS and EMP data. The fine continuous line connects the <0.10 mm (2d-1) and >0.25 mm (2d-2) splits recovered after wet grinding of the fourth fraction handpicked from the >0.50 mm grain size. The fine dashed line connects the two fractions [phengite (1) and phengite (2d-2)] that were subjected to the wet-grinding and sieving procedure, and correspond to the purest fractions analysed |
References cited
Vannucci R., Piccardo G.B., Rivalenti G., Zanetti A., Rampone E., Ottolini L., Oberti R., Mazzucchelli M., Bottazzi P.: Origin of LREE- depleted amphiboles in the subcontinental mantle, Geochim. Cosmochim. Acta, 59 (9), (1995), 1763-1771.
Ottolini L.: Accurate SIMS analysis of Ca in olivine based on high-energy doubly charged secondary ions, J. Anal. At. Spectrom., 17 (2002), 280-283.
Di Vincenzo G., Tonarini S., Lombardo B., Castelli D., Ottolini L.: Comparison of 40Ar-39Ar and Rb-Sr Data on Phengites from the UHP Brossasco-Isasca Unit (Dora Maira Massif, Italy): Implications for Dating White Mica, J. Petrol., 47, (2006), 1439-1465.